I’ve been thinking a lot about
disruption
lately. The modern business-jargon use for the word bothered me the first time I saw it. The term seems to lose most of its negative connotations as users try to express their ideas about radical change but “for the better.” Look up
disrupt
in a thesaurus, however, and you’ll find that its synonyms are all unfortunate in nature.
Coming from the Latin
dis
- (“apart”) and
rumpere
(“to break”),
disrupt
literally means “to break apart.” It first was used medically to refer to tissue-laceration injuries, then gained more general traction in the 1600s. The Online Etymology Dictionary has a fun graph showing the use of
disruption
from books going back to 1800. Its frequency remained below two in a million words until about 1940, then leapt to seven in a million between 1960 and 1980. Contrary to my sense of it in recent years, the word’s use has remained relatively steady since then.
I think that what’s bothered me has been the gleeful marketing materials tout...
The biotechnology market is enduring historically low access to venture capital (VC), private equity (PE), mergers and acquisitions (M&A), and initial public offerings (IPOs). As a result, biotechnology companies must chase after the fleeting funding opportunities out there today (
1
). By prioritizing the right marketing strategies immediately, companies can maximize available VC, PE, and M&A opportunities, enabling them to establish long-term success and focus on science.
According to Santollani, the bulk of biotechnology costs go to research and development (R&D), employee compensation, scientific advisory boards (SABs), contract research organizations (CROs), rent, and legal services (
2
). But that assessment did not discuss marketing as a budgetary consideration, which is an important topic to many biotechnology companies. For organizations that don’t sell products, allocating a percentage of revenue to marketing does not apply. However, for those that do, I recommend that 8–10% of a company’s budge...
Effectively managing deviations is fundamental to ensuring product quality, regulatory compliance, and patient safety. Intricate processes are involved in deviation management, from the initiation phase, where incidents are swiftly evaluated, to the comprehensive investigation of root causes. Through a systematic examination of impact assessment, deviation investigations, and implementation of corrective and preventive actions (CAPAs), I seek to provide a thorough understanding of the critical components that contribute to a robust deviation-management system. Here I focus on the pragmatic methodologies, challenges, and strategic considerations that characterize efficient deviation management within the biopharmaceutical industry, emphasizing the importance of timely actions and continual improvements to establish robust quality systems.
I
ncident Discovery and Immediate Actions
Traditionally, incident discovery begins when a deviation initiator opens a deviation report. That person collaborates with a qu...
The popularity of OpenAI’s ChatGPT program exemplifies society’s growing awareness of the remarkable power of artificial intelligence (AI) and machine learning (ML). Digital transformation is both democratizing access to information and helping users to translate it into knowledge. AI and ML can augment our ability to collect and analyze data in ways similar to how robots increase our ability to examine and relocate physical objects.
Biopharmaceutical professionals also are recognizing AI’s/ML’s potential for applications along the pharmaceutical life cycle. Drug-development activities are becoming more complex and difficult as the amount and variety of process and product data grow. But AI is demonstrating its ability to handle tasks such as analysis of massive data sets, multivariate analysis, decision-making, issue identification, process automation, and system modeling and control.
We humans are good at addressing creative tasks and applying abstraction to complex problems. On the other hand, people t...
Genome-modified hematopoietic stem and progenitor cell (GM-HSPC) therapies represent a significant frontier in the realm of personalized medicine, holding the promise of targeted interventions for a spectrum of disorders far beyond hematological conditions. Development of these therapies is tied intricately to the rich history of and advancements in hematopoietic stem cell (HSC) grafts, which have served as the cornerstone for our understanding of hematopoiesis and bone-marrow transplantation (
1
).
The journey toward gene-edited stem cell therapies began with the foundational work of E. Donnall Thomas and colleagues during the 1950s and 1960s. Their studies enabled the refinement of protocols for bone-marrow transplantation and demonstrated regenerative potential of HSC grafts transplanted into recipients, thereby reconstituting their blood and immune systems (
2
). The 1970s brought a shift toward allogeneic HSC transplants, necessitating further refinements in transplantation techniques and immunosuppr...
Process capability often serves as a metric for quantifying biomanufacturing-process performance. The term refers to the extent of variation in measurable property
X
of a given biological product made by a given process relative to the width of that property’s specification limits. Thus, process capability provides insight into how consistently a manufacturing process generates products that meet specifications. In other words, the higher the process capability is, the lower the probability is for an out-of-specification (OoS) outcome.
Evaluating process capability is particularly important in biopharmaceutical manufacturing. Multiple analytical results (often with multiple replicates) are generated routinely for release of drug-product batches — hence the need to ensure consistent process performance and product safety and efficacy.
Definitions:
Statisticians working in industry and academia have proposed several process-capability indices (
1
). Equations 1–3 list the most basic indices for a process...
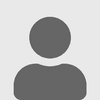
+1Visual inspection for equipment cleanliness is an important element of cleaning validation and monitoring. Multiple regulations, guidances, and standards address expectations for cleaning (
1–4
). Visual inspection may be used to verify attainment of health-based cleaning limits as described in guidances from the International Council for Harmonisation of Technical Requirements for Pharmaceuticals for Human Use (ICH) (
5
) and Pharmaceutical Inspection Convention and Pharmaceutical Inspection Co-Operation Scheme (PIC/S) (
6
) so long as the conditions of Questions 7 and 8 from the associated question-and-answer documents are met (
7, 8
).
Visual inspection offers several advantages over cleaning verification based on analytical testing (see the “Advantages and Disadvantages” box on the next page) (
9, 10
). But several published studies have documented widely varying detection limits for visual inspection for small molecules ranging from 0.4 μg/cm
2
to >10 μg/cm
2
(
1, 11–14
).
Validation engineers freq...
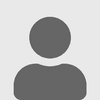
+3Regulations in the United States and elsewhere require that 100% of parenteral drug products be inspected for defects and that released drug products be “essentially free” or “practically free” of visible particulate matter. Single-use systems (SUS) and equipment used in bioprocessing must meet the level of cleanliness required to enable biopharmaceutical manufacturers to meet current good manufacturing practice (CGMP) and pharmacopoeial requirements regarding particulate matter.
USP <788> and USP <790>
Historically, biomanufacturers have asked SUS suppliers to “meet USP <788> specifications for particulate matter” (
1
). However, as discussed in detail in
a recent BPI article
, the US Pharmacopeia’s (USP) chapter <788> is misapplied to SUS (
2
). The “Particulate Matter in Injections” chapter describes test methods and limits for subvisible particulate matter in parenteral drug products only — not SUS. Adaptation of those test methods to the measurement of particulate matter in SUS requires addition of ...
There is nothing more exciting in the biopharmaceutical industry than the rapid advancements in cell and gene therapies (CGTs). As scientists develop new technologies and solutions, developers work to translate research and learnings from academia into tangible, life-saving cures. Successfully developing new therapies hinges on extensive collaboration, not only among academia and industry, but also among manufacturers and their quality-control (QC) partners. Collaboration aids discoveries that pioneer the future of medicine and shortens the learning curve for all stakeholders through sharing knowledge from research, trials, and experiences. Immediately after companies secure funding, they consult their QC partners to help navigate the regulatory landscape with the vision and agility necessary to bring revolutionary offerings to the market safely and effectively.
My company works with CGT manufacturers to create robust safety-testing plans to support development. No two therapies, testing strategies, or co...