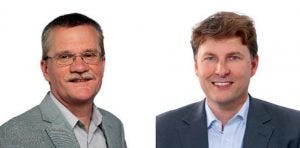
Armin Hauk and Alexander Trappe
Extractables and leachables (E&Ls) must be addressed in material and process validation programs. Extractables are compounds that can be extracted from a material in the presence of solvents with varying polarity under extreme conditions. Materials manufacturers should make extractables guides available to end users. Leachables are compounds that migrate from a material in the presence of an actual formulation under normal process operating conditions. Extractables information can be helpful as a basis for evaluation of potential process-equipment–related leachables (PERLs)testing.
However, extractables data and potential leachables must be correlated carefully. After over 20 years of E&L testing, the industry has found very few leachables–drug interactions. With a fully elucidated extractables profile, it is possible to extrapolate leachbales. In most single-use systems (SUSs), only a low number of PERLs become true leachbales and only at very low concentrations.
In container–closure systems, however, specific formulations can affect the type and quantity of leachables. So pharmaceutical manufacturers must demonstrate that the leachables do not contribute to adverse toxicological effects in the drugs they make. Regulatory guidances indicate that it is best to test for leachables with an actual formulation. Based on applied risk assessment, an array of analytical techniques can be used to detect and identify compounds released from polymer-based materials, even in the most complex product formulations.
Armin Hauk is principle scientist in extractables and leachables, and Alexander Tappe is head of regenerative medicine at Sartorius Stedim Biotech. In fall of 2019, we discussed the overlap of those two topics, and their combined answers are included herein.
Making Extrapolations
How are extractables data used in estimating product and patient exposure? Extractables results are valuable for predicting or forecasting the identity, range, and maximum concentration of PERLs and leachables in a drug substance (DS) or drug product (DP). Consequently, extractables data enable assessment of potential risks for process performance, product quality, and patient safety (1).
Assessment of patient safety has become quite sophisticated. In classical toxicology assessments, a toxicologist estimates potential patient exposure based on extractables data and compares the results with permitted daily exposure (PDE) or tolerable daily intake (TDI) values to determine a safety margin. For unidentified extractables, that comparison is made directly with threshold of toxicological concern (TTC) values, which can be found in a number of publications, including the ICH M7 guideline.
Methods for assessment of process performance and/or product quality are not as well developed. Only a very few such effects of PERLs have been described in scientific literature (2). Although in that case there was an impact on process performance, we are not aware that any drug product was affected. And the predictive determination of PERL concentration in process streams is not yet well established. Common “worst-case” approaches — simply scaling up and cumulating extractables data — overestimate the potential PERL load so completely that such values cannot be used in a reasonable risk assessment on process performance and/or product quality.
For cell and gene therapies (CGTs), it is recommended to use the same principle approach in assessing process performance, product quality, and patient safety. But we have to admit that the potential influence of PERLs on cell viability and integrity is of much higher relevance here than in classical biopharmaceutical production. We can argue that most CGT applications will have low and infrequent doses, so direct patient exposure to leachables in such drug formulations is less critical than it can be in other pharmaceutical applications. However, the influence of PERLs on therapeutic cells is of highest interest. Effects of leachables on cell viability and cell integrity are linked directly to patient-safety considerations in CGT and thus need to be well understood.
How can extractables data be obtained for single-use devices of different sizes and for device combinations? Doing testing for all devices on the market is effectively impossible because of the overwelming number of SUS, so we must rely on data from product families and scale the results. In general, two methods are applied:
For short–contact-time materials, you can scale extractables based on the product-contacting surface area of the single-use system (SUS). Under diffusion-controlled conditions, the migrating amount of extractables or leachables is directly proportional to the exposed surface area. Sartorius has published on that, and it works fine even for complex devices (3). The obtained scaling results are valid only for the time point at which extractables data were available. If you need to scale a device and extrapolate to other time points (temporal scaling), then you must use migration calculations.
For long–contact-time materials, scaling can be based on partition calculation after equilibrium is reached between SUS material and the contacting liquid phase.
How can extractables data be extrapolated toward potential leachables? What scaling methods apply? A number of methods work on different levels of mathematical complexity. Scaling methodologies described above can be used, for example, to extrapolate extractables data. PERLs can be extrapolated from extractables data using partition and migration calculations, and the dynamic nature of a process step can be taken into account with dynamic box modeling. The “fate of leachables” in combined process steps and/or entire processes can be calculated using modular dynamic box modeling.
All those methods are based on physical principles that are known, well established, and valid for the phase transfer of compounds (from solid to liquid). Because the models use physical principles, they are are considered to be mechanistic. To use such models in CGT requires extending them to include cells in the calculations by considering the biological matrix as a kind of “third phase.”
Mitigating the Risks
What are some approaches to quantitative mitigation in assessing single-use systems? The most widely discussed mitigation concept in the SUS area — assessing PERLs and finally leachables in a process — is the “proximity to patient concept.” The closer to the final DP a SUS is used, the higher its criticality; SUSs used far upstream from the DP are of less criticality. This concept is reasonable, but it is just a qualitative methodology that cannot be used to quantitatively mitigate risk.
Other mitigation concepts are based on the observation that, for instance, protein capture or buffer exchange with tangential-flow operations can separate PERLs from product streams (by removing them with the rinsing- or permeate fractions). Such mitigation concepts are quantitative, but we cannot be 100% certain that they work with all potential PERLs. Experimental results must prove that they will before you can use them in a process validation, for example.
Another quantitative approach would be to develop dynamic process models that describe leachables load along a process from the sources (SUS materials) by migration calculation and including all possible sinks associated with a process (e.g., phase separation, adsorption dilution, and so on). PERL forecasts from such “fate-of-leachables” models can be both qualitative and quantitative and come quite close to reality (4).
Comparing Results
How does data interpretation differ between container–closure systems (CCS) and single-use bioprocess equipment? Data interpretation for CCS and SUS are quite similar, although experimental conditions in the associated extractables studies are based on different preconditions.
With CCSs you can anticipate that at the end of the product shelf-life leachables could approach or reach equilibrium concentrations: an equilibrium between CCS material and the liquid formulation. So forced, exaggerated, or even exhaustive extractables experiments using shredded materials and reflux extraction would be justified as appropriate. But for SUSs, all published protocols and proposals tend to simulate somehow typical process conditions of use. Results from such simulation studies are related to applied, dedicated, experimental conditions — making extrapolation to other conditions difficult or even impossible. Often, the experimental conditions in such studies do not even provide a full overview of potential extractables.
Thus, the main difference in evaluating CCS and SUS extractables is that for the former we rely on comprehensive information from extractables studies, and for the latter an evaluation of SUS extractables often is based on limited information from simulation studies. This is why we at Sartorius Stedim Biotech prefer to conduct true extractables studies that give a full and comprehensively elucidated overview about all potential extractables (5). For data evaluation toward process conditions, we recommend using physical methods to extrapolate or forecast to potential PERLs in spatial and temporal dimensions.
Most proposed standardized extractables protocols for simulating the use of SUS in classical biopharmaceutical processes are justifiable only partly to CGT applications. The reason is simply that all those protocols use simulation liquids, whereas in CGT we need to get additional insights regarding PERLs released from SUS and effectively available for interacting with cells.
What other concerns arise in tackling these issues for regenerative medicine? Within this space, some applications are close to classical biopharmaceutical applications. For example, viral-vector production for ex-vivo or in-vivo gene therapy often uses similar process strategies and technologies as those used in vaccine manufacturing. Therefore, similar risk-assessment strategies apply, as do reductions of E&Ls by individual unit operations. Consider a medium exchange from growth to production medium during upstream processes or in purification steps such as affinity chromatography or crossflow filtration. Effectively PERLs can be removed from such process fluids before final fill.
Ex-vivo gene therapies (e.g., many autologous cell therapies) provide an additional layer of safety. PERLs present after final filling will not be subjected to patients directly, but rather will be process contaminants in cell expansion and transduction that eventually generates gene-modified cells as a drug product. Those unit operations will reduce the PERL concentration coming from the vector manufacturing even further.
Of course, single-use assemblies used during biomanufacturing of cell-based drugs pose a source of E&Ls for both gene-modified and unmodified cells. Here you need to take a closer look at your process strategy. In gene-modified cell applications such as chimeric-antigen receptor (CAR) T cells, you will often find media-exchange processes that remove activation and/or transduction reagents from the cell environment.
Perfusion processes are popular here, allowing continuous removal of PERLs. In addition, several process strategies include affinity-based cell-selection steps during or after cell expansion, which presents another opportunity for removal of contaminants by wash buffers. Wash steps also are performed during centrifugation (e.g., with counterflow centrifuges). All these steps can remove hydrophilic PERLs.
The one big difference from traditional bioprocessing is that cells are not contaminants in regenerative medicine and not removed from the process stream. Any hydrophobic PERLs that stick to cell membranes and get removed in classical bioprocessing will remain on cell-based drugs in regenerative medicine applications. Fortunately, hydrophobic substances represent just a fraction of PERLs, and the capacity of cell surfaces to bind them probably is limited. We are aware of those differences, however, and have started investigations to get a better understanding of cell interactions with E&Ls (6).
Can you comment on the ELSIE database (www.elsiedata.org/elsie-database) and its potential value? ELSIE is a nonprofit organization founded by members of the pharmaceutical and biopharmaceutical industry, and since 2018 it also has included members from the supplier industry. ELSIE established a forum for the industry to discuss assessment of extractables data and harvest toxicological data for a wide range of extractables from CCSs and SUSs. Today, the database contains nearly 500 entries relevant for risk assessment. The big advantage of such a database is that it serves as a single point of truth in extractables risk-assessment exercises. Currently ELSIE is engaging suppliers to bring their knowledge on material-related extractables into the database. Sartorius fully supports this approach, and we are convinced that a database linking materials with anticipated extractables and toxicological information is of high value for all stakeholders in this area.
What is missing from the database are PDE/TDI values for extractables, but hopefully in time ELSIE can provide such data as well. It is clear that an industry-wide, approved set of PDE and TDI values for extractables would reduce necessary efforts in an E&L assessment to a minimum if such a data set could serve as single point of truth.
We would love to see ELSIE further extend its database to physicochemical parameters necessary for calculating, predicting, and modeling PERLs and leachables. Those parameters also could be helpful in toxicokinetic evaluations. In the future, ELSIE might not only harvest toxicology information used in patient safety assessments but also data on interactions of PERLs with cells used in the CGT area.
References
1 Li K, et al. Creating a Holistic Extractables and Leachables (E&L) Program for Biotechnology Products. PDA J. Pharm. Sci. Technol. 69(5) 2015: 590–619; doi:10.5731/pdajpst.2015.01073.
2 Hammond M. et al. Identification of a Leachable Compound Detrimental to Cell Growth in Single-Use Bioprocess Containers. PDA J. Pharm. Sci. Technol. 67(2) 2013: 123–134; doi:10.5731/pdajpst.2013.00905.
3 Pahl I, et al. Using Extractables Data of Sterile Filter Components for Scaling Calculations. PDA J. Pharm. Sci. Technol. 73(6) 2019: 523–537; doi:10.5731/pdajpst.2018.00964.
4 Hauk A, et al. On the “Fate of Leachables” in Biopharmaceutical Up-Stream and Down-Stream Processes. Single-Use Technologies II: Bridging Polymer Science to Biotechnology Applications. Proceedings of Biopoly II, Tomar, Portugal, 7–10 May 2017.
5 Pahl, I. et al. Development of a Standardized Extractables Approach for Single-Use Components: General Considerations and Practical Aspects — A Manufacturer’s Perspective. BioProcess Int. eBook 23 October 2018.
6 Paudel K, et al. Quantitative Characterization of Leachables Sinks in Biopharmaceutical Downstream Processing. Eur. J. Pharm. Sci. in press 2019; doi:10.1016/j.ejps.2019.105069.
For more information, contact Armin Hauk, Sartorius Stedim Biotech GmbH, August-Spindler-Straße 11, 37079 Göttingen, Germany; [email protected].