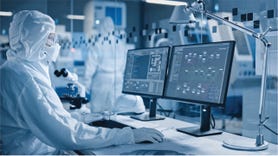
The advent of advanced therapy medicinal products (ATMPs) has ushered in a new age of healthcare by providing curative solutions to some of the world’s most debilitating diseases. ATMPs represent an inflection point in our industry’s ability to improve patient treatment. A November 2023 report states that 34,400 patients were treated with chimeric antigen receptor (CAR)-T cell therapy, which is being hailed as the “holy grail” of cancer therapies because, in some cases, it can achieve complete response (CR) rates of >80%. (1, 2). Drug developers reached this milestone thanks to decades of research that broadened our understanding of the underlying biology, immunology, and genetic engineering that drive new therapies toward favorable clinical outcomes.
But the ATMP revolution in medicine presents a unique set of challenges, especially regarding advanced manufacturing, logistics, storage, and data analysis. Groundbreaking manufacturing and digital technologies are now integral to ATMP development. Such complex and sensitive medicines demand critical data analysis and engineering oversight, and that presents both risks and opportunities for transformative healthcare. By adopting new technologies, developers ensure scalability of ATMPs and thus improve patient access to them.
A Convergence of Capabilities
As digital and medical technology begin to merge, experts agree that we are blurring the boundaries between biomanufacturing and supply-chain logistics (3). ATMPs are hyper-delicate, temperature-sensitive, and often personalized, thus necessitating unfailing adherence to current good manufacturing practice (CGMP) and good distribution practice (GDP). That makes a high degree of automation, rigorous raw-materials management, real-time cold-chain visibility, and design for manufacturing (DfM) techniques minimum requirements for the industry. It also brings an emphasis on reducing cost of goods sold (CoGS) to enable widespread patient access.
ATMPs use mechanisms of action (MoA) not typically found in conventional therapy and require highly specialized manufacturing. ATMP manufacturing encompasses a spectrum of methods for conferring unique properties on immune cells or vectors by harnessing or augmenting cellular-signaling pathways. That often comes through gene-editing or gene-engineering approaches that include the following:
• surface-receptor modification, as with CAR T-cell therapies
• use of cellular machinery to express proteins not normally produced by cells, such as in nucleic-acid–based therapies
• assemblage of cells/scaffolds into larger structures used in regenerative-medicine applications, as in tissue engineering.
In most cases, manufacturers source starting materials from patients and then perform ex vivo modification and/or analytical testing. The resulting materials are used to produce an ATMP through bioreactor cultivation or biochemical synthesis. That general process yields highly efficacious drugs that target unhealthy cells and tissues with extremely high specificity — and often with tolerable levels of off-target toxicity and minimal adverse events. Entirely new classes of drugs have arisen from these approaches. ATMPs are described broadly as cell therapies, gene therapies (e.g. those based on adenoassociated virus (AAV) vectors), gene-modified cell therapies (e.g. CAR-T therapies), nucleic-acid drugs (e.g. mRNA therapies including personalized cancer vaccines, or PCVs), tissue engineered products, clustered regularly interspaced short palindromic repeats (CRISPR)-edited therapies, and even radioligand therapies and other nuclear medicines. After decades of research and advances, these new classes are changing medicine fundamentally.
Enhancing the Supply Chain To Reach Patient Populations
Regulatory bodies are approving new modalities for human use in larger patient populations, which demands strict considerations for manufacturing. ATMPs are made with biological materials that are modified or transformed to confer new properties. Such materials are susceptible to biological variability that can arise from donors. Moreover, finished products are sensitive and must reach patients within strict temperature controls and time constraints.
The cells, nucleic acids, vectors, and reagents used to manufacture ATMPs must be processed expertly, stored, and transported at ultra-low or cryogenic temperatures to retain their potency. Typically, liquid-nitrogen vapor keeps cells at or below –150 °C, whereas dry ice keeps viral vectors, gene therapies, and nucleic-acid therapies at or below –60 °C (4). In many cases, raw materials are subject to the same GMP and GDP standards as the drug products that they are used to create. Cell lines, blood-derived products, protein supplements, and viral and nonviral vectors each have transport and storage requirements that further complicate logistic, storage, and manufacturing considerations.
ATMPs have achieved unprecedented success, especially given their relative infancy. The gene therapy Casgevy (exagamglogene autotemcel) was approved in January 2024 to treat sickle-cell anemia, joining six US Food and Drug Administration (FDA)–approved CAR T-cell therapies that treat hematologic malignancies. Regenerative approaches such as tissue-engineered skin grafts for burn victims also attest to the transformative power of ATMPs (5).
Disease survivors Emily Whitehead and Jennelle Stephenson exemplify the effectiveness of ATMPs in continuing to live free from their afflictions — cancer and sickle-cell disease, respectively — for years after treatment (6, 7). Such patient experiences showcase the long-term efficacy of such products. To further illustrate the field’s rapid ascent, consider the ATMP market valuation of US$8 billion in 2022. Experts predict a compound annual growth rate (CAGR) of 13%, leading to a valuation of $24 billion in 2032, tripling the market size over the span of a decade (8).
Although ATMPs promise personalized and potentially curative treatments, their developers face critical challenges in scaling production to meet demand. A key obstacle lies in the inherent complexity of development. Unlike mass-produced chemical drugs, many ATMPs are customized for patients. Individualized therapies cannot be mass-produced and often are incompatible with industrialized workflows. Balancing cell expansion, vector production, and ex vivo manipulation requires specialized, functionally closed systems managed by highly skilled personnel. Those difficulties increase costs and complicate scaling; however, the growing field of allogeneic therapies could help (Figure 1).
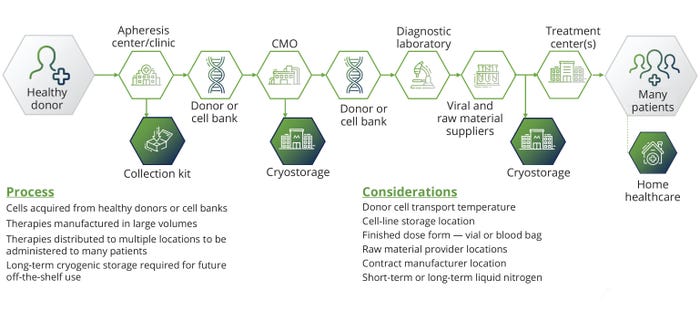
Figure 1: The stages of allogeneic therapy development.
Streamlining Processes for Worldwide Availability
Before ATMPs become mainstream, researchers must resolve bottlenecks with upstream and downstream processing. In current upstream processes, the cost of goods (CoG) to manufacture ATMPs is prohibitively high. Developers need to select donors for allogeneic ATMPs carefully and then monitor and control immune responses to prevent the unfavorable outcomes associated with poor long-term persistence and low yields. Likewise, isolation or selection steps are necessary to purify cell populations of interest, but those steps decrease viability or recovery of cell populations depending on the techniques used.
Developers also must consider the source and grade of the ingredients that they use to produce ATMPs. Such materials bring stability, reliability, and cost challenges. Patient materials needed for process development, manufacturing, and testing are often difficult to obtain. It is critical that companies achieve flawless supply and delivery of ATMP-production components. Knowledgeable logistics partners are vital to maintaining success throughout that process.
After perfecting sourcing and transport fundamentals, developers must assess risks and mitigate delays that arise when working with contract development and manufacturing organizations (CDMOs) that offer process development and GMP manufacturing capabilities. Viral-vector availability and logistics variables including cold-chain reliability, chain of custody/ identity issues, visibility, and losses due to poor package integrity or transport conditions can cause significant problems. To address those, manufacturers should consider novel packaging solutions with longer validation times. Nevertheless, further innovation is needed to meet industry demands (9).
Industry Innovations: New technologies are being assessed to determine the best manufacturing processes. The presence of stagnation points in fluid paths and leachables and extractables in film materials damage cell viability and function, leading to excursions from established critical-to-quality (CTQ) attributes of a final product. Engineers have improved agitation systems with rocking platforms, vertical wheels, and conventional impellers, as well as gas-exchange systems such as spargers. Those modifications improve culture intensification but might introduce problems from shear sensitivity, clumping, or inadequate dissolved oxygen (DO) rates. More uncertainty arises from biological variation in cells among patients. In autologous ATMP production, a patient’s disease state can affect cell expansion significantly (Figure 2). Allogeneic processes involve healthy donors, but major differences in cell productivity arise from inherent biological differences that necessitate donor screening.
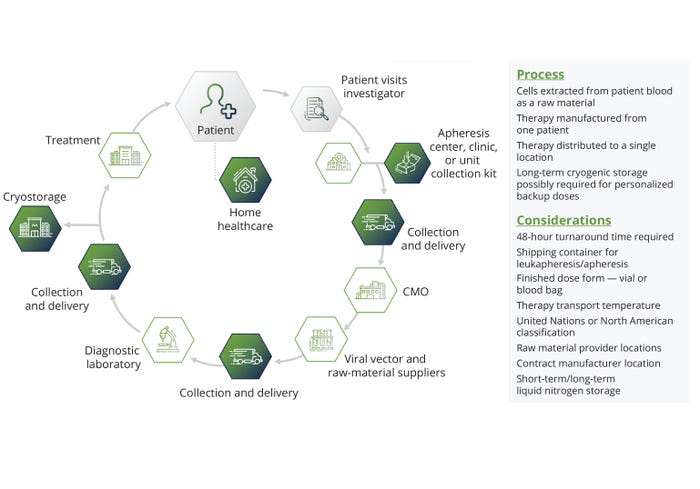
Figure 2: The stages of autologous therapy development.
Inefficiencies from enriched media and reagents can cause pitfalls and lead to high costs. Researchers are investigating how to design such media to improve productivity and lower cost through rigorous design of experiments (DoE) approaches and metabolomics-driven discovery of novel media candidates with cell-type and application-specific formulations development.
Several new players are innovating downstream-processing technology using an array of techniques such as counter-flow centrifugal elutriation (CCE) and tangential-flow filtration (TFF). When manufacturers use magnetic beads to separate and activate cell populations such as T cells and natural-killer (NK) cells, debeading is often required, but that can cause product losses. Feeder systems also add complications, requiring additional oversight for irradiating, maintaining, removing, and assaying unwanted cell populations. The advent of beadless activation and separation reagents has helped, but many manufacturers continue to rely on bead- and feeder-based methods during upstream processing.
Unfortunately, losses are inevitable when scaling. For example, low yields can occur with each subsequent wash during a final media exchange, but washes are necessary to remove excipients and ancillary materials during production. The numerous steps involved in downstream processing always result in losses. Viral-vector production, terminal filtration, sterilization, and media exchange all happen before final formulation. But the greater those hold points are studied and allayed, the better process control manufacturers will have over the final drug product infused into a patient.
Choosing the Right Strategy: In addition to navigating upstream and downstream bottlenecks, ATMP sponsors consider whether allogeneic or autologous approaches are more sustainable and scalable. Allogeneic therapies are considered the most viable for scalable mainstream application. They have immense promise for off-the-shelf, on-demand administration of therapies to patients, but also carry the potential for immune-mediated rejection resulting from graft versus host disease (GvHD) or host versus graft disease (HvGD). Moreover, a lack of fit-for-purpose reagents and bioreactors to manufacture such therapies at scale has hampered their progress. The screening and selection of healthy donors continue to be critical for driving the expansion of parental cells. That said, developers can use pluripotent stem cells (PSCs) as a parental cell source and combine them with techniques to mute key immunogenic cell-surface receptors to solve some concerns. Improvements in manufacturing and clinical-trial design will help ensure that the most appropriate donor and patient populations are selected for further investigation and that their therapeutic benefits are maximized in vivo.
Optimizing Storage for Safeguarding Products
Once process yields are maximized, cryopreservation practices must account for cell-type sensitivities. For example, some populations of cells, such as NK cells, are especially sensitive to cryopreservation (10). Fortunately, certain tools help to maintain cell viability both ex vivo during manufacturing and in vivo upon patient infusion. Useful tools include controlled-rate freezers and novel cryopreservation formulations that eliminate the need for components such as dimethyl sulfoxide (DMSO). Despite the availability of those tools and reagents, a lack of cell-type specific data across the patient population persists, raising another obstacle to driving decisions around parameters such as cooling-curve profiles.
Similar issues arise when manufacturers choose vials or bags to freeze their products. Users must decide how to address the risks associated with leachables and extractables and consider how best to contain products transported with liquid nitrogen and/or dry ice (11). Many autologous therapies use bags to enable transporting of the infusion volumes required in a minimal number of shippers (as few as one per patient), but there are exceptions. Allogeneic therapies — and the working cell banks from which they are manufactured — typically are packaged into vials and transported en masse with bulk shippers for larger patient populations.
Finally, maintaining visibility of raw materials and final drug products during transport is key for ATMP manufacturers and infusion centers. To meet that need, stakeholders use end-to-end track-and-trace technology with specialized data loggers that monitor key parameters such as temperature, light, shock, tilt, and global positioning system (GPS) coordinates during transport. The use of round-the-clock control towers is the gold standard to ensure that therapies reach their final destinations, offering manufacturers and logistics providers a means of intervention in cases of severe disruption (12). Manufacturers need to address difficulties through automation, novel sensing systems, and loggers that provide real-time location and status data. Applying such solutions will help enable ATMP manufacturing and logistics to be independent of human intervention.
Vision for the Future
Although ATMPs are revolutionary, best practices for their development and manufacture still are being determined. The FDA recently issued a new box label warning for CAR-T products to highlight their potential risks. It was deemed necessary after a review found a small number of secondary cancers in patients who received CAR-T cell therapies (13, 14). Scientists are working to determine whether nonviral vectors can be used in ATMP production to eliminate safety concerns. Nonviral vectors such as mRNA-loaded lipid nanoparticles (LNPs), prime and base editing approaches such as CRISPR-based editing, and nonviral chemical modifiers are still in development, representing an opportunity for researchers to use novel methods for cell modification. That said, the tide is turning. The Casgevy product represents the FDA’s first approval of a CRISPR-modified gene therapy (15). That landmark decision granted credibility to the idea of using nonviral editing methods.
Looking to the Future: Digitization, automation, and predictive modeling of manufacturing workflows are positioned to accelerate the development of new modalities. The power of artificial intelligence (AI) and machine learning (ML) is reducing trial times by as much as 75% while increasing accuracy for targeted indications (16). Sponsors have poured investments into AI technology over the past year (17). The benefits of in silico systems are demonstrated further in strategies that use digital twins, wherein a manufacturing process is modeled and tracked with a set of input parameters. Those results drive decisions independent of the need for cell sampling or process analytical technology (PAT). Manufacturers use analytical and sensing technology throughout a supply chain to improve transport visibility, product integrity, and cold-chain logistics. Visibility tools such as temperature loggers and tilt, shock, light, and vibration sensors help safeguard ATMP integrity. Smart software and application programming interfaces (APIs) help to integrate and leverage existing solutions across the bioprocessing ecosystem.
Key players are developing streamlined manufacturing methods to reduce timelines for autologous therapies. Novartis, for example, developed its T-Charge platform to reduce manufacturing times to two days. BMS, the University of Pennsylvania, and Gracell Biotechnologies all are following suit with their own respective platforms (18–20). Scientists are evaluating whether such technologies eliminate the need for complex manufacturing workflows and whether patients should take on the role of bioreactors by harnessing the innate potential of cells to proliferate in vivo after ex vivo gene modification and activation.
Without considering the impact to genotype and phenotype, indefinite cell expansion to a achieve a desired cell number poses a risk to cells and patients. Cells can become terminally differentiated over time, losing their potency and “stemness.” In response, researchers are further motivated to eliminate complex manufacturing and manipulation steps (21). Although shortened manufacturing processes appear to represent faster time to treatment, some industry experts have expressed skepticism that such changes cut total vein-to-vein time (22). The logistics surrounding such approaches would not change fundamentally and would require more frequent placements, returns, and live shipments to prevent shipping bottlenecks and preserve the turnaround time (TAT) for patients. Thus, additional work is needed to understand the benefits and disadvantages of using such approaches.
Some manufacturers seek to use (AI) to predict bioreactor output trends, find areas for improvement, and establish upper and lower bounds on donor-to-donor variability. AI adoption improves understanding of trends in big data that surround cell-therapy production bottlenecks depending on biological factors. AI also enables manufacturers to appropriately correct for those trends by adjusting manufacturing parameters, such as agitation rates, gas-exchange rates, and time in culture.
The holy grail of ATMP manufacturing is complete process automation. That would enable remote management of manufacturing and cold-chain logistics using real-time sensing tools to monitor cell phenotypes and other metabolic parameters, such as lactate production and glucose consumption as surrogates for cell sampling of biomass. Finally, manufacturers need improved packaging and closed-loop solutions for cold-chain logistics, including reliable sensing technologies such as data loggers that enable real-time visibility of product stability. Manufacturers also need appropriate storage facilities to maintain products in ultracold environments prior to patient administration. There is a major need for CGMP depots with advanced centralized monitoring system (CMS) capabilities. Those should include alarms and push notifications on freezers and refrigerators that trigger in the event of temperature excursions. It is also important to have operator safety features such as low-oxygen alarms to detect abnormal off-gassing events in rooms containing liquid-nitrogen systems (23).
As more clinical and commercial drug products are made available to patients, biomanufacturer needs will increase. It is important to anticipate storage needs and retain samples for quality control (QC) so that ongoing treatments and manufacturing activities continue to run smoothly. There must be cooperation among stakeholders across industry, academia, hospitals, and specialty logistics providers to achieve a common vision of improving development and manufacturing of advanced therapies. Only then can we ensure safe, efficacious, and expeditious treatment for patients.
References
1 Liu A. As FDA Probes CAR-T Safety, Expert Meeting for Bristol’s Abecma Could Serve as Key Guidepost. Fierce Pharma 29 November 2023; https://www.fiercepharma.com/pharma/fda-inspects-car-ts-safety-risk-adcomm-bristols-abecma-could-offer-guidepost.
2 Dagar G, et al. Harnessing the Potential of CAR-T Cell Therapy: Progress, Challenges, and Future Directions in Hematological and Solid Tumor Treatments. J. Transl. Med. 21(449) 2023; https://doi.org/10.1186/s12967-023-04292-3.
3 Boldt V, Krause S, von Wintzingerode F. Continuing the Conversation for Better ATMP Development. PDA Letter 2023; https://www.pda.org/pda-letter-portal/home/full-article/continuing-the-conversation-for-better-atmp-development.
4 Meacle F, et al. Key Considerations of Cell and Gene Therapy Cold Chain Logistics. Cell Gene Ther. Ins. 2(2) 2016: 223–236; https://www.insights.bio/cell-and-gene-therapy-insights/journal/article/501/Key-considerations-of-cell-and-gene-therapy-cold-chain-logistics.
5 Approved Cellular and Gene Therapy Products. US Food and Drug Administration: Rockville, MD, 2024; https://www.fda.gov/vaccines-blood-biologics/cellular-gene-therapy-products/approved-cellular-and-gene-therapy-products.
6 Moore A. Emily Whitehead, First Pediatric Patient To Receive CAR T-Cell Therapy, Celebrates Cure 10 Years Later. The Children’s Hospital of Pennsylvania: Philadelphia, PA, 11 May 2022; https://www.chop.edu/news/emily-whitehead-first-pediatric-patient-receive-car-t-cell-therapy-celebrates-cure-10-years.
7 Cunningham D. FDA Approves First Human Gene Editing Therapy for Sickle Cell Disease. United Press Int. 8 December 2023; https://www.upi.com/Health_News/2023/12/08/sickle-cell-gene-editing-treatment-approved/4521702058989.
8 Global Advanced Therapy Medicinal Products (ATMPs) Market Size To Reach USD 24 Billion in 2032. Reports and Data: New York, NY, 18 April 2023; https://www.reportsanddata.com/press-release/global-advanced-therapy-medicinal-products-market.
9 This Is Drug Distribution. Evolved. Marken: Durham, NC, 13 June 2023; https://www.marken.com/learning-center/this-is-drug-distribution-evolved.
10 Domogala A, Madrigal JA, Saudemont A. Cryopreservation Has No Effect on Function of Natural Killer Cells Differentiated In Vitro from Umbilical Cord Blood CD34+ Cells. Cytotherapy 18(6) 2016: 754–759; https://doi.org/10.1016/j.jcyt.2016.02.008.
11 Packaging and Conditioning. Marken: Durham, NC, 2024; https://www.marken.com/capabilities/packaging-conditioning.
12 Cell and Gene Control Towers. Marken: Durham, NC, 9 January 2024; https://www.marken.com/capabilities/cell-gene-therapy/cell-therapy-management-control-towers.
13 Liu A. Cell Therapy Bigwigs Endorse CAR-T in Nature Medicine Article amid FDA Safety Probe. Fierce Pharma 2024; https://www.fiercepharma.com/pharma/cell-therapy-bigwigs-endorse-car-t-nature-medicine-article-amid-fda-safety-probe.
14 Amorosi D. FDA Requires Boxed Safety Warning for All CAR T-Cell Therapies. Healio 23 January 2024; https://www.healio.com/news/hematology-oncology/20240123/fda-requires-boxed-safety-warning-for-all-car-tcell-therapies.
15 FDA Approves First Gene Therapies To Treat Patients with Sickle Cell Disease. US Food and Drug Administration: Rockville, MD, 8 December 2023; https://www.fda.gov/news-events/press-announcements/fda-approves-first-gene-therapies-treat-patients-sickle-cell-disease.
16 Borfitz D. AI Transforming Real-World Clinical Development. Clin. Res. News 21 February 2023; https://www.clinicalresearchnewsonline.com/news/2023/02/21/ai-transforming-real-world-clinical-development.
17 Pecci A. 5 Noteworthy Pharma AI Investments in 2023. PharmaVoice 19 December 2023; https://www.pharmavoice.com/news/pharma-ai-investment-pfizer-sanofi-novartis/702882.
18 Kerber A. What’s the Future of Cell Therapy at BMS? Bristol Meyers Squibb: Lawrence Township, NJ, 8 November 2023; https://www.bms.com/life-and-science/science/discussing-the-future-of-cancer-treatments-using-cell-therapy.html.
19 Begun C. Penn Researchers Shorten Manufacturing for CAR T Cell Therapy. Penn Med. News 29 March 2022; https://www.pennmedicine.org/news/news-releases/2022/march/penn-researchers-shorten-manufacturing-time-for-car-t-cell-therapy.
20 Yang J, et al. Next-Day Manufacture of a Novel Anti-CD19 CAR-T Therapy for B-Cell Acute Lymphoblastic Leukemia: First-in-Human Clinical Study. Blood Cancer J. 12(7) 2022; https://www.nature.com/articles/s41408-022-00694-6.
21 Salz L, et al. Culture Expansion of CAR T Cells Results in Aberrant DNA Methylation That Is Associated with Adverse Clinical Outcome. Leukemia 37(9) 2023: 1868–1878; https://www.nature.com/articles/s41375-023-01966-1.
22 Plieth J. Ash 2022 — Fast Production Fails To Cure Car-T’s Problem. Evaluate 2022; https://www.evaluate.com/vantage/articles/events/conferences-trial-results/ash-2022-fast-production-fails-cure-car-ts.
23 Temperature Data Monitoring for Freezers (up to –20 °C). Elpro: Buchs, Switzerland, 2024; https://www.elpro.com/en/equipment-monitoring/freezer-monitoring-systems.
Rohin K. Iyer, PhD, is senior director of cell and gene operations, and Robert Brackner is global content manager, both at Marken, 1009 Slater Road, Suite 120, Durham, NC 27703. For inquiries, please contact [email protected].