“We are witnessing one of the most significant paradigm changes in oncology drug development, with some new types of immunooncology compounds inducing unprecedented increases in survival in certain solid and liquid tumor indications.” —Jagath Reddy Junutula (Cellerant Therapeutics) and Hans-Peter Gerber (Pfizer) (1)
An antibody–drug conjugate (ADC) for cancer treatment needs four things to succeed clinically: It needs to target the right antigen using the right antibody, with the most powerful cytotoxin attached by best linker option. These features combine to endow the resulting conjugated product with therapeutic safety and efficacy.
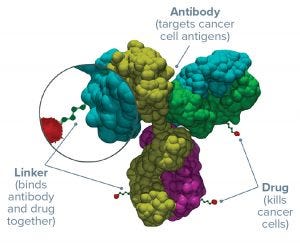
Figure 1: Three parts of an antibody–drug conjugate
Antigens: Determining which receptor to target is the first step in ADC development. The best options are expressed highly and consistently on the surface of a number of tumor cell types — but absent from normal tissues (or at least expressed at very low levels) — and won’t be downregulated after initial ADC treatment. If tumor cells stop expressing that antigen in reaction to their first exposure to a drug, then subsequent treatments won’t have the same cytotoxic effect. Tumor-specific antigens are the ideal choice, although so far most ADCs have targeted antigens that are only tumor “associated” (e.g., HER2 in breast cancer).
Antibodies: Human or humanized antibodies, bispecifics, and perhaps antibody fragments can form the mechanism to bind to a chosen antigen. IgG monoclonal antibodies (MAbs) are the most common choice. Some antibodies have their own immunogenic cytotoxic effects, and some can by their size or other aspects prevent internalization of an ADC complex into cancer cells — potentially exposing nearby tissues to drug effects. Representing by far the largest part of an ADC drug substance, a MAb must be well understood to be used for this purpose.
Linkers: The molecular linkage between an antibody and its cytotoxic payload plays a vital role in determining the safety, specificity, potency, and activity of an ADC. The number of drug molecules that get attached to each antibody (expressed as the drug/antibody ratio, DAR) makes a big difference as well. Ideally, linkers are stable in a patient’s bloodstream but break down easily inside target cells. More than anything else, advancements in linker chemistry have made ADCs a viable therapeutic reality.
Cytotoxins: For both chemical and biological reasons, only small amounts of a cytotoxic drug can be expected to reach the intended site of action — but more with an ADC format than traditional systemic chemotherapy. The chosen payload must be both stable and highly potent. Antibodies offer a means of using the most potent cytotoxins that are too dangerous for systemic delivery — enhancing the therapeutic efficacy of chemotherapy while reducing systemic toxicity.
To succeed commercially, an ADC also needs to meet a few other requirements related to quality and safety. Some stem from the molecular classes listed above, and some entail aspects of quality by design (QbD) and risk management. Workers must be kept safe from highly potent drugs; products must be kept safe from potential contamination. Drug-substance characteristics must be controlled, methods of documenting that control must be validated, and cost of goods (CoG) cannot be an afterthought.
FULFILLING POTENTIAL
So far, five ADCs have been approved for oncologic indications in the US and European markets: Adcetris (brentuximab vedotin) from Seattle Genetics; Kadcyla (ado-trastuzumab entansine) from Genetech/Roche; Besponsa (inotuzumab ozogamicin) and Mylotarg (gemtuzumab ozogamicin) from Wyeth Pharmaceuticals, a subsidiary of Pfizer; and Lumoxiti (moxetumomab pasudotox-tdf) from Innate Pharma and AstraZeneca. Nearly 200 others are in development, over 10% of those at phase 3 or better as of early 2018 (2). Acute adverse effects manifested with the earliest products in development, making it clear that ADC sponsors would need to address concerns with immunogenicity, linker stability, target antigen choice, DAR, and dosage/bioavailability issues.
Nonhuman antibodies were used in the very first ADCs, with associated immunogenicity problems (e.g., generation of human antimurine antibodies) that are familiar to MAb developers. Immunogenicity is more likely to create trouble for the drug itself rather than the patient who receives it, but it needs to be prevented either way. If a person’s immune system attacks a MAb product carrying a cytotoxic payload, there’s a chance that those cytotoxins could be released into the patient’s bloodstream. And with the high potency of modern ADCs, that can be a real problem. Development of humanized and then fully human antibodies has gone a long way toward lessening these immunogenicity concerns, both with MAb therapeutics themselves and their ADC derivatives. Toxicity concerns can arise with different linker chemistries, as well: The first ADC linkers were supposed to be released under acidic conditions in or near tumors; unfortunately, those early linkers weren’t as trustworthy as promised, proving to be unstable in circulation.
Early ADCs had to be administered at high doses. Low MAb concentrations in blood plasma could not provide appropriate bioactivity, especially with just ~1% of an injected dose able to reach its tumor target (3). And to deliver and release its payload into a cancer cell, an ADC first must bind to its target antigen on the surface of that cell. Early ADCs targeted antigens that did not always express at high enough levels. The resulting limitations on delivery could not provide enough cytotoxins to kill tumor cells. And not all targeted antigens could internalize ADC complexes, anyway. As with many things in biology, this great idea of “magic-bullet” MAb delivery of cytotoxins to malignant cells wasn’t quite so easy as at first it had seemed.
The Next Generations: Many problems with those first-generation ADCs were eliminated in the development of second-generation products. Premature drug release was solved through use of different linkers: e.g., Genentech/Roche’s noncleavable thioester linker and Seattle Genetics’s cleavable valine–citrulline linker. Newer ADCs carry more potent cytotoxins such as monomethyl auristatins, which can be 1,000× stronger than older options. The new drugs can be too toxic for ordinary chemotherapy use, in fact, but safer for patients when carried by MAbs for targeted delivery.
Second-generation ADCs have their own issues, most notably related to product heterogeneity. Chemical conjugation typically involves lysine or cysteine MAb residues, resulting in up to eight payload molecules per MAb (averaging three or four). After the chemical reaction step, a final ADC drug substance can comprise a mixture of unconjugated, partially conjugated, and overconjugated antibodies. Conjugation site also has been shown to influence potency, stability, and pharmacokinetics.
It’s not easy to explain heterogeneity to regulators, especially when unconjugated and drugconjugated antibodies compete for antigen binding sites — thus diminishing bioactivity overall. On the other hand, overconjugation can destabilize and cause ADC complexes to aggregate, thus leading to possible immunogenic responses and nonspecific toxicity as well as shortening the product’s lifespan in circulation. Heterogeneous ADCs thus have limited efficacy and even might induce drug resistance in some tumors.
In evaluating its own linker technology, Innate Pharma developed a dye-based assay for assessing the aggregation of ADCs (4): “Stability of an ADC depends largely on the number of toxins conjugated to the antibody and the lipophilic nature of linkers used to conjugate those toxins. Applying the dye resulted in the successful prediction of the propensity for aggregation of ADC products, thereby validating the coupling technology as an ideal platform for development of stable, robust ADC products.”
Cleavable linkers are considered to be more broadly effective than noncleavable options, mainly but not only because even when whole ADCs are not fully internalized by cancer cells, they can still take action. Free drug molecules released from MAbs can permeate cell membranes on their own, especially with highly expressed cancer-cell surface receptors just waiting to grab them.
Current work is taking us into an era of third-generation ADCs. Site-specific conjugation has been introduced to produce more homogeneous ADCs with well-characterized DARs and cytotoxicities. Ideally this approach will provide a single-isomer ADC with a uniform DAR value — although that image of perfection is not easy to achieve. Most companies thus far are happy being able to narrow their design space to a couple/few documented isomers (with one clearly dominant) and an acceptable DAR range (with a nice, steep bell curve).
Many of the newest ADCs are based on bioengineered antibodies that contain site-specific amino-acid, glycan, or peptide “tags” for linking. Another approach is Abzena’s “Thiobridge” technology that connects payload drugs to the interchain disulfide bonds in the antibody hinge region (3). Non-native amino acids also can be engineered into the antibody structure. Creative Biolabs, for example, has developed a conjugation platform called PtLnX that uses bis(ethylenediamine) platinum chloride as a linker core for ADC development. The technology forms stable linkages with nitrogen-containing organic alkalines (e.g., N-heteroaromatic and N-aliphatic amine) in antibody residues such as histidine.
Building on lessons learned, ADC developers are going after rationally chosen antigens, using novel linker chemistries, and investigating payload options to enhance the targetability and therapeutic efficacy of these drugs. Improved product understanding will help companies best define their manufacturing strategies and process/product quality attributes for regulatory filings based on risk management and quality by design (QbD). This should improve clinical results, expand therapeutic options, and lead to more product approvals in the near future.
MANUFACTURING PROCESS ISSUES
ADCs are proven to be a viable product modality. The technology works. But as New Jersey company Immunomedics learned earlier this year, proving that your drug works is only half of the equation. Based on data integrity and manufacturing issues, the company’s sacituzumab govitecan ADC for metastatic triple-negative breast cancer (TNBC) was not approved after priority review. The humanized IgG1 antibody targets a cell-surface glycoprotein expressed in >90% of such cancers with the antineoplastic drug SN-38 (an active metabolite of irinotecan chemotherapy with 1,000× more activity than irinotecan itself). It’s a much-needed treatment for a frequently deadly cancer.
“We believe that sacituzumab govitecan has the potential to be a viable treatment,” said Immunomedics president and chief executive officer Michael Pehl in a company statement. “The issues related to approvals in this CRL [complete response letter} focus only on chemistry, manufacturing, and controls and do not require generation of new clinical or preclinical data. We will request a meeting as soon as possible to fully understand FDA requirements and approval timetables, and we will work closely with [the agency] to bring this important drug to patients as soon as possible.”
The FDA had given this ADC priority review based on data from an ongoing phase 2 study showing that the product offered a significant improvement over the (currently dismal) standard of care, and industry analysts were predicting its market value to be over US$1 billion. With approval, the ADC would be the first and only treatment for metastatic TNBC, a notoriously fatal condition. A phase 3 clinical study is in progress now, and the company is planning to test this product for other metastatic cancers as well.
As mentioned above — and not to imply that it is the problem in the Immunomedics case — product heterogeneity has been a major problem in ADC process development. But it’s not the only challenge.
Creating best-in-class ADCs by producing homogeneous molecules has tremendous potential to optimize clinical results by improving efficacy and product safety. Homogeneity in itself is insufficient. Systematic design of these complex molecules will require real drug-discovery–based reiterative design and development of structure– activity relationships to guide design of optimized molecules. Biochemical protein expression may be one such solution. (5)
Cleaning and waste handling are critical factors in ADC manufacturing (6). Cytotoxic waste cannot be disposed of into sewers directly. Such materials must be collected and handled properly. Here, single-use technologies offer some help by eliminating the need for cleaning. But disposable materials also require appropriate waste logistics.
Employees must be protected from highly potent cytotoxins, too. Whereas biologics facilities have overpressurized cleanrooms to protect their product streams from contamination, ADC facility designs uses underpressurized spaces with isolators and fume hoods to prevent employee exposure and release of cytotoxins to outside environments. The complexity and internal contradictions of ADC manufacturing makes outsourcing an attractive option for many companies. But data management and project oversight then become key. Partnerships between biotechnology companies with MAb expertise and drug companies with chemotherapy capabilities have shown the most success.
DELIVERING ON THE PROMISE
“The complex heterogeneity of cancer cells and their increased mutation rates remain a challenge for the efficacy of ADCs. . . . New generations of ADCs are developed based on advances in antigen identification, linker technology, and warheads. Like with other targeted therapies, combination of different modalities may prove superior. The evaluation of combinations of ADCs with other antibodies, small-molecule inhibitors, and even immune checkpoint inhibitors is in very early stages. Such approaches are destined to provide exciting clinical data in the future with the expectation to not only prolong survival, but also improve quality of life of cancer patients.” —Cécile Chalouni (Genentech/Roche) and Sophia Doll (Max Planck Institute) (7)
Regulatory Questions: Although the ADC future looks bright, challenges remain. Because this therapeutic class is relatively new, regulators have yet to put forth much specific guidance. The possible route of administration for a drug product in a specific patient population affects its formulation and presentation. Controlling product characteristics such as DAR continues to present some difficulty in manufacturing, as does development of analytical methods to support process and product development.
Meanwhile, the pharmacokinetic and metabolic aspects of ADCs are highly complex. Characterizing their absorption, distribution, metabolism, and excretion (ADME) is difficult because the underlying mechanisms are not fully understood. Developers need to assess the relationships between pharmacokinetics and pharmacodynamics of individual ADCs.
For those ADC developers targeting orphan diseases, preclinical testing often is complicated by limited understanding of their causes and molecular mechanisms and a rarity of animal models. Developing suitable in vitro safety models to is difficult even with big-data approaches, which much include factors such as route of administration, product formulation, and cell-surface interactions.
Finally — and despite all the promise and solid science of the technology — ADCs in clinical trials do suffer from a similarly low “rate of complete response” to that of other cancer treatments. Dose-limiting toxicities also continue to present problems, indicating the need for more study. Patients selected for high expression of tumor-specific antigens should show complete responses to ADC treatment, with substantially less systemic toxicity than would be expected from the cytotoxin alone. Unfortunately, that is not always the case, and researchers continue to investigate possible reasons why. Combining immunotherapy and ADCs may be the answer. Some studies have shown substantial improvements in response rates with combination therapy (8).
REFERENCES
1 Junutula JR, Gerber H-P. Next-Generation Antibody- Drug Conjugates (ADCs) for Cancer Therapy. ACS Med. Chem. Lett. 7(11) 2016: 972–973; https://europepmc.org/ articles/pmc5108033.
2 Pearce M. ADCs Have Finally Come of Age. EPG Online 14 February 2018; https://www.epgonline.org/uk/ horizons/antibody-drug-conjugates–adcs-.html.
3 Waterman S. Technology Advances Enable Creation of Better ADCs. BioProcess Int. October 2014; https:// bioprocessintl.com/2014/technology-advances-enablecreation- better-adcs.
4 Schneider N, et al. Predicting Aggregation Propensity and Monitoring Aggregates in ADCs. BioProcess Int. October 2014; https://bioprocessintl.com/2014/predictingaggregation- propensity-monitoring-aggregates-adcs.
5 Hallam T, Bender J, Newell B. Fine-Tuning ADCs for Best-in-Class Therapeutics. BioProcess Int. October 2014; https://bioprocessintl.com/2014/fine-tuning-adcs-bestclass- therapeutics.
6 van de Leur A, Eppink M. Development and Biomanufacturing Strategies for Next-Generation Antibody–Drug Conjugates. BioProcess Int. December 2018; https://bioprocessintl.com/manufacturing/emergingtherapeutics- manufacturing/development-andbiomanufacturing- strategies-for-next-generation-antibodydrug- conjugates.
7 Chalouni C, Doll S. Fate of Antibody-–Drug Conjugates in Cancer Cells. J. Exp. Clin. Cancer Res. 37, 2018: 20; https://www.ncbi.nlm.nih.gov/pmc/articles/ PMC5802061.
8 Vankemmelbeke M, Durrant L. Third-Generation Antibody Drug Conjugates for Cancer Therapy: A Balancing Act. Ther. Deliv. 7(3) 2016; https://doi.org/10.4155/tde- 2016-0002.
9 Sharma V. CPhI Annual Report 2018: ADCs Growth Driven By Lack of In-House Facilities, Oncology and Integrated CDMOs. Pharm. Outsourcing 10 October 2018.
Cheryl Scott is cofounder and senior technical editor of BioProcess International, PO Box 70, Dexter, OR 97431; 1-646-957-8879; [email protected].