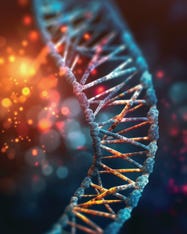
New genetic-engineering techniques, analytical methods, and laboratory technologies are helping to elucidate the inner workings of mammalian and other cells to an extent once never imagined. Cell biologists and process engineers now have an unprecedented ability to refine and control performance in cell cultures. Along with advanced information technologies (ITs), those successes already are changing how biopharmaceutical companies around the world undertake cell-line development (CLD). This past March 2024 at BPI West in San Diego, CA, an entire conference track was devoted to reports of such progress.
Keynotes and Featured Presentations
Key talks from “big-pharma” and academic speakers highlighted advances in digitalization, manufacturing technologies, and genetic modification. In “Digital Transformation: Accelerating Process Development and Enhancing Control Strategies,” Tiago Matos (associate principal scientist at Merck) explored the concept of digital twins for biomanufacturing facilities, highlighting the current challenges to their use — including infrastructure requirements — as well as their potential for continuous processing, design space definition, and control-strategy implementation. To be truly useful, virtual representations must reflect real-world biomanufacturing conditions and equipment functions accurately.
Nuno Fontes (head of global biologics development at Bayer US) emphasized what’s required to commercialize advanced therapies with “Advances in Process and Manufacturing Technology Platforms for the Industrialization of Allogeneic Cell Therapy.” Chemistry, manufacturing, and controls (CMC) remains a critical bottleneck in getting cell therapies to patients on a global scale, he said. Developers need technology platforms that accelerate industrialization to enable global access. Fontes touted the value of partnering among academic and industrial institutions to develop flexible platform solutions for cell-therapy bioprocessing.
Genetic Engineering: Nathan Lewis (professor at the University of California, San Diego) brought a key theme of the program to light in “Engineering Out Metabolic Barriers in CHO Cells.” Until recently, most gains in productivity for bioprocessing — e.g., enhancements in cell growth, viability, and resource allocation — have come through clone selection, media screening, and process optimization. Those gains have been impressive, but Lewis said that systems and synthetic biology now provide diverse new tools for taking cell and process enhancement to the next level. He focused on metabolic barriers to productivity in recombinant-protein production.
Two key such barriers in mammalian cell culture are concurrent production of lactate and ammonium (NH4). Lewis showed how they could be eliminated to improve cellular productivity. Lactate dehydrogenase (LDH) activity interferes with glycation patterns. The possible solution, Lewis indicated, would be to knock out related genes, thus “unplugging a sensor and removing a feedback loop.” In experiments selecting for clones with knocked-out LDH genes, the resulting cells did not produce lactate, but they grew better than wild type cells did. NH4 is another metabolic by-product seldom considered in cell-line engineering. The Lewis Laboratory used a triple-gene knock-out used to eliminate NH4 production.
Jacqualyn Schulman (senior scientist in CLD and ‘omics for biologics development at BMS) also reported on gene knock-out techniques in “Engineering CHO Cells To Improve a Post-Translational Modification for a Bispecific Antibody.” Although some posttranslational modifications (PTMs) are necessary for protein function, she said, some others can lead to undesired effects, such as increased immunogenicity, loss of efficacy, and decreased stability. Schulman’s team used genetic engineering of CHO cells expressing a bispecific antibody (bsAb) to knock-out key genes involved in a problematic PTM: sulfotyrosine modification (1).
The BMS team determined that the gene encoding for tyrosylprotein sulfotransferase (TPST) would be the best target for knock-out and used clustered regularly interspaced short palindromic repeats (CRISPR) technology in a proof-of-concept study. Knocking out both TPST forms decreased tyrosine sulfation by ~46%, thus eliminating the sulfation problem. And it did not compromise cell growth, viability, titer, or product quality.
Takeshi Omasa (professor in the graduate school of engineering at Osaka University) reported on development of new cell lines in “Next-Generation Chinese Hamster–Derived Host Cell: Development and Characters.” Working with academic and industry collaborators, his laboratory in Japan established a CHO-MK line of ovary cells, a CHL-YN line of lung cells, and a CHK-Q line of kidney cells. The ovary and lung cell lines are adapted to serum-free media and boast doubling times of ~10 hours — nearly twice as fast as those of commonly used CHO cell lines — with comparable glycan profiles. Also adapted to glutamine-free conditions, the open-source CHL-YN line is available from the RIKEN cell bank (2). The CHO line is available commercially from Chitose Laboratory.
Session Presentations
Genetic Engineering: Several presenters reported on knock-out work and targeted-integration (TI) techniques. In “Generation of Fucosyltransferase 8 Knock-Out CHO-K1 Host Cell Lines,” Tiffany McLamarrah (senior scientist at Sanofi) showed how glycoengineering enhances the potency of therapeutic antibodies. Those without core fucose residues have strong antibody-dependent cellular cytotoxicity (ADCC) at relatively low doses because they present no gamma receptor IIIa for serum immunoglobulin G (IgG) to bind to and inhibit their function.
Advances in gene editing provide a means to edit CHO genes with few off-target effects. The Sanofi team applied Cas-CLOVER fully dimeric nuclease technology (Demeetra) to generate several glycoengineered CHO-K1 cell lines by knocking out fucosyltransferase 8 (FUT8) genes. Resulting clones yielded 100% afucosylated antibodies, and whole-genome sequencing demonstrated no off-target effects.
In “Sialic Acid Modulation for Enzyme Fusions Using Combined Approach of Cell Line Engineering and Cell Culture Process Development,” Yashas Rajendra (associate director and principal scientist at Denali Therapeutics) explained that sialylation is important for therapeutic glycoproteins such as enzyme fusion molecules, which have short half-lives. In CHO cells, sialylation is modulated through gene overexpression or media supplementation. Denali used a combined approach with transposon-mediated pools for reliable and rapid translation to clones specific to modulation of sialylation.
In “CHO Cell-Based Targeted Integration Platform for Accelerated Antibody Development,” Yuansheng Yang (senior principal scientist at A*STAR in Singapore) said that antibody discovery typically includes either in vivo or in vitro work followed by production in CHO cells. To improve efficiency at that stage, Yang’s team developed an integration platform for simultaneous display and secretion of antibodies. “This platform enhances antibody development by generating high-affinity, developable antibodies and supporting rapid production for preclinical and clinical studies.”
The process generates stable pools in about three weeks, with selection of production clones (from 10–20 to the top three) in about five weeks for stability testing, process development, and generation of a master cell bank. The A*STAR team obtained titers >5 g/L in seven weeks and took eight weeks to produce a research cell bank (RCB). Yang reported that the bsAb-producing clones are genetically stable over >90 generations.
A senior scientist at Genentech/Roche, Zion Lee discussed the “Impact of Gene Copy Number and Configuration on Antibody Production in Targeted Integration CHO Cell Lines.” Increasing transgene copy numbers often is presumed to enhance transcriptional activity and product yield. Lee said that TI can be used to investigate the relationships among gene copy number, mRNA abundance, product yield, and product quality.
“Incrementally elevating the gene copy numbers of both IgG heavy chains (HCs) and light chains (LCs) concurrently resulted in the attainment of plateaus in mRNA levels and product titers,” he explained, “notably occurring beyond four to five gene copies integrated at the same TI site.” Maintaining a fixed copy number while varying the position of genes within the vector changed LC/HC and mRNA ratios, which subsequently influenced product titer. Manipulating the LC/HC gene ratio by introducing surplus LC gene copies heightened LC mRNA expression and reduced expression of high–molecular-weight species.
Lee noted that the effects of excess LC on product titer differed by molecule of interest. Strategic use of polymerase chain reaction (PCR) tags enabled precise quantification of transcription from each expression slot within the genetic vector used, helping to identify the most and least expressive slots. “Collectively, these findings significantly enhance our understanding of stable antibody production in TI-CHO cell lines.”
From Pfizer, Kathryn Beal (principal scientist in process development) reported that “The Impact of Expression Vector Position on Transgene Transcription Allows for Rational Expression Vector Design in a Targeted Integration System.” The goal was to maximize performance of a dual site-specific integration system for CHO expression of complex biotherapeutics. “We investigated positional effects within expression vectors by engineering nucleotide polymorphisms to quantify transcripts from each vector position. These learnings allowed for rational expression-vector design, resulting in optimized productivity and product quality.”
Pfizer’s platform features two independently addressable “landing pads” with a unidirectional Bxb1 bacteriophage serine DNA recombinase for targeted genetic integration to generate phenotypically and genetically stable clones. Beal said that it expresses multispecific molecules with high productivities and product percentages. Studies of vector composition showed that a LL-HH configuration provided increased productivity of all mAbs tested.
Software and Hardware: Microfluidics, next-generation sequencing (NGS), automation, and artificial intelligence/machine learning (AI/ML) were featured prominently in other track presentations.
Lina Chakrabarti (associate director of R&D at AstraZeneca) described her experience in “Simplifying CHO Cell Line Generation with High Probability of Monoclonality Using Microfluidics Dispensing as an Alternative to FACS.” Her team found the Namocell Pala system to be easier to use and its single-cell deposition efficiency to be comparable with that of a fluorescence-activated cell sorting (FACS) system. She was pleased with the “high probably of monoclonality,” comparable growth profiles, and production capability of the resulting clones. The microfluidic system significantly reduced scientist time required for getting results as well as the cost of consumables and maintenance. So AstraZeneca has found it to be a suitable and reliable replacement.
Valerie Komatsu (process development senior scientist at Amgen) also used “Microfluidic Chip–Based Single-Cell Cloning and Clone Screening To Enrich for High Quality Manufacturing Cell Lines.” She said that CLD campaigns can be resource intensive, with hundreds of clonal cell lines first expanded and then screened for productivity, product quality, and stability profiles. Amgen uses the PhenomeX Beacon nanofluidic platform for culturing thousands of clonally isolated cells simultaneously on a single chip. Spotlight assays (also from PhenomeX, formerly Berkeley Lights) based on a fluorescently conjugated probe against the crystallizable fragment (Fc) quantify steady-state secreted protein levels for individual clones. However, Komatsu’s team wanted to determine whether on-chip secretion measurements would be predictive of future productivity under conditions of manufacturing scale.
“We established a representative and measurable model system to assess clone-screening efficiency of Beacon CLD workflows,” she said. “Our process eliminates poor candidate clones during single-cell cloning and enriches for top-producing cell lines with desirable manufacturability profiles. Our CLD platform integrated with the ability to leverage assays for clone characterization enables early identification of high-quality manufacturing cell lines.”
The CLD team established a representative and measurable pseudopool model system to assess single-cell cloning and clone-screening efficiency, then used that to evaluate for single-cell cloning and clone selection. “Clones with superior performance can be identified from thousands of cell lines evaluated at the point of single-cell cloning,” Komatsu said. “Our process allows enrichment for top-performing cell lines and eliminates poor candidate clones as assessed by both small-scale clone screening and a [Sartorius] Ambr 15 scale-down model.”
NGS was the focus of presentations from Seagen (now part of Pfizer) and Takeda Pharmaceuticals. In “Next-Gen Sequencing Platform and Applications in Cell-Line Development,” Ying Shen (director of CLD at Takeda Pharmaceuticals) reported on incorporation of sequence-variant evaluation by NGS into the CLD process for protein biologics and retroviral vectors. For clone selection and quality control, Takeda uses an in-house–developed Illumina MiSeq method to eliminate clones that show gene-of-interest (GoI) sequence variance. Shen said that NGS also could be used for detection of potential adventitious pathogens.
In “Early Development of Next-Generation Sequencing for Clone Selection and Genetic Characterization,” Sofie O’Brien (senior scientist in bioprocess development at Seagen) reported that her company has implemented NGS for clone selection and is exploring it to complement or replace other testing. Her team developed a high-throughput targeted-sequencing platform to evaluate clonally derived production cell lines. It provides early data on transgene sequence, integrated vector integrity, and genomic integration sites for improved clone screening and characterization.
Seagen used the platform to assess monoclonality through integration analysis of subclones. The method begins with thawing cells from an MCB and passaging them to identify integration sites present, followed by subcloning with a single-cell printer and reviewing the monoclonality of wells that show outgrowth. Clones that originate from single cells are subcloned further into 24–deep-well plates before high-throughput genomic DNA extraction using an automated bead method. Results from a quantitative PCR (qPCR) monoclonality assay (with primers designed to amplify identified integration junctions) go through statistical analysis to calculate confidence of clonal derivation.
“NGS has the potential to address multiple platform needs with one in-house assay,” said O’Brien. DNA sequence-variant analysis provides high-throughput early screening of clones before extensive characterization would be possible. Seagen found that analyzing targeted sequence data can provide comparable information to that derived from standard genetic-characterization methods. Targeted sequencing of development banks then can be used as an early screen for genetic stability before lead-clone selection. And integration sites from targeted sequencing can be used as primers to provide evidence of clonal derivation even for legacy cell lines.
Two suppliers — one of services, the other of technology — featured applications of modern IT in CLD. HaeWon Chung (senior synthetic biologist at Asimov) presented “Integrating Synthetic Biology and Computer-Aided Design To Advance Biologics Production in CHO Cells.” Transfection methods and fed-batch processing have brought impressive advances in CHO cell culture, production, and stability. But Chung said that genetic-vector design typically still takes a “one-size-fits-all approach.” A therapeutic molecule’s coding sequences are inserted into a fixed plasmid, which contains a small set of legacy genetic components. Such a rigid approach can lead to suboptimal expression and thus impede the clinical development of mAbs and their complex derivatives.
Instead, Asimov’s CHO Edge system tailors a vector for each molecule in development to optimize its expression. The suite integrates a CRISPR-edited glutamine synthetase (GS) knock-out CHO host with a hyperactive transposase, libraries of characterized genetic elements to control cellular functions, and computational tools for rational vector design and multiomics analysis. Chung said that those tools enable development of high-copy, high-expression producer lines with long-term stability; fine-tuning of heavy- and light-chain expression and GS selection; and ML-based optimization for increased translational efficiency, secretion, and cell growth. Case studies highlighted the optimization of expression for mAbs and bsAbs at 6–10 g/L with consistent expression stability over four months (from transfection to RCB). This model-guided process can be accessed through licensing or as a service.
Michael Chen (chief executive and cofounder of Great Bay Bio) described another broad platform in “AI + Site-Specific Integration Platform: AlfaCell.” His company uses AI to replace repetitive screening. Chen said that the AlfaCell site-specific integration platform speeds up CLD to improve bsAb development, producing monoclones within six weeks after transfection under a 5-g/L titer guarantee (with >80% correct pairings). Great Bay Bio combines that platform with cell-culture media development to accomplish process development within two to three months. That’s followed by mAb sequence screening, in vitro studies, and animal studies within the time span that’s usually required for mAb sequencing alone (six months). Chen says that his company cuts overall timelines from mAb sequence to manufacture roughly in half using AlfaBody (for sequence generation), AlfaDAX (molecule optimization), AlfaCell (CLD), AlfaMedX (media development), and AlfaOPA (media optimization) platforms.
Workflows: Using technological advancements as described above, biopharmaceutical companies continue to refine and improve their CLD workflows. One approach that is receiving more attention than it has in some years is early production by stable pools rather than clonal cell lines. For example, Yves Durocher (mammalian cell expression section head of at the Human Health Therapeutics Research Center, part of Canada’s National Research Council (NRC)) described “Rapid Development of Trimeric SARS-CoV-2 Spike-Based Vaccine Candidates Using Stable CHO Pools.” He said that stable CHO pools were used during the pandemic to manufacture SARS-CoV-2 neutralizing antibodies for early clinical trials. And he presented data supporting use of the same approach to manufacture complex trimeric spike antigens. “Spike quality attributes are maintained when deriving stable clones from the pool.” Data showed that CHO pools also could be used to manufacture highly immunogenic SARS-CoV-2 spike-based enveloped virus-like-particles (VLPs).
As Durocher illustrated, the typical workflow is five weeks for pool generation and establishment of a research cell bank, 15–20 weeks for stable clone development, and 20 weeks for GMP MCB generation and release to manufacturing for phase 1. NRC’s stable-pool generation and production process uses MSX selection to go from transfection to MCB in ~5 weeks, then under two months for expansion, production, and purification of GMP material. His team first accelerated the work by eliminating the step of stable-clone development, which saved 15–20 weeks, then moved “at pandemic speed” to shorten the GMP step from 20 down to 8 weeks.
“Stable CHO pools are producing very high levels of the SARS-CoV-2 trimeric spike protein,” he concluded, “with quality attributes comparable to [material] produced by clones.” The pools are stable for >30 generations and amenable to robust upstream processes in bioreactors. “This is a rapid and cost-effective platform to manufacture spike antigens (and other biologics) for early clinical evaluation.”
CHO cells may dominate, but they’re not the only cells used in biomanufacturing. Yi Liu is a scientist and team leader of CLD for the vaccine production program at the Vaccine Research Center (VRC), a division of the National Institute of Allergy and Infectious Diseases, which is part of the US National Institutes of Health. In “Development of Stable Pool Expression Platform in HEK293 Cells,” Liu explained that limitations in CHO cells prompted the VRC team to explore other expression systems, such as human cell lines. CHO cells have difficulty synthesizing and secreting some complex proteins, leading to low productivity, growth restriction, expression instability, and overall low resistance to stresses, all of which incur high production costs.
“We developed a stable-pool expression platform using HEK293 [human embryonic kidney] cells.” Through a rolling project pipeline, VRC screened for optimal medium and transfection conditions, compared selection markers and pressures, and evaluated different expression-vector systems (cotransfection, linearized vectors, and the “Sleeping Beauty” transposon system). The CLD team compared transient and stable-pool expression of the same protein in CHO-DG44 and HEK293 cells, focusing on timeline and production levels. HEK293 stable pools generated using a linearized vector in the phleomycin D1 selection system gave the best product yield in the shortest time.
Panel Discussion
One highlight of the CLD conference track was a roundtable panel discussion moderated by Ken Lee (director of bioprocess technologies and engineering in biopharmaceuticals development R&D at AstraZeneca) with panelists Sampath Kumar (senior director and head of CLD in biologics process development at Takeda Pharmaceuticals), Saurabh Sen (associate director of CLD in the genomic medicine unit’s CMC group at Sanofi), and Lina Chakrabarti of AstraZeneca. To start the discussion, Lee posed questions to both the panelists and the audience, beginning with “How many cell lines should you bring out of development?”
Panelists described the possibility of moving forward with less-than-ideal cells to produce early clinical trial material, then plan to continue development behind the scenes. Through cell-line optimization, companies can increase expression titers for commercial manufacturing and switch to a final optimized cell line when needed. This approach could speed early development and defer some cost to later phases — once a product has been deemed to be worthy of more investment. It relates to the “fail-early” rule of thumb in an industry that faces notoriously high product-attrition rates.
One audience member wondered about the risk/benefit ratio and return on investment (RoI) for such strategies, and another attendee suggested that drug modality plays a role. Some complex molecules (e.g., mAb derivatives) require additional cell lines for derisking along the way. Lee added that choosing between random and targeted integration of transgenes also could figure into the equation.
Chakrabarti pointed out that each random integration yields more clonal variation and can lead to much higher titers ultimately. Targeted integration simplifies screening and gives more predictable results but limits the range of mutations that can arise in the resulting cell pools. She said that 10 g/L is now standard for mAb programs beginning with 192 clones at small-scale screening, then moving to a pool of the top 15–24. Random integration can increase productivity by multiples rather than the mere percentages achievable using targeted and transposon-based engineering.
Diversity is limited with transposase technology, Sen agreed, such that 4–5 g/L productivity is the likely limit from duplicate/triplicate pools — but that is arrived at quickly, with good product quality. But new analytical technologies are streamlining the means of screening, thus improving the chances for random integration to produce very high-titer clones. And Kumar explained that transposase-based pools cannot provide such early titers because of the limited number of gene copies that integrate (due to the limited places they can insert in a cell’s genome).
An audience member asked what the “acceptable” expression titer for mAbs is now. Panelists agreed that it’s ~6–10 g/L after process optimization. Chakrabarti cautioned that product variants can complicate matters for some complex molecules, causing product-quality concerns when they’re produced at higher titers. The attendee agreed, noting that titer should be less of a concern than product quality partly because it’s easier to get. With bsAbs, for example, the correct pairing is key, making site-specific integration a necessity. Limiting the numbers of mispaired molecules saves time and effort in later development.
Another audience member mentioned that site-specific integration is “still a new technology and getting better.” One big-pharma company is “seeing 7–8 g/L from such work.” And for multispecific antibodies, modulation of expression vectors can help to predict and provide the correct moiety ratios without the need to screen too many clones. Thus, Chakrabarti said, “depending on modality, we decide which way to go.” And Kumar agreed. “The technology is improving as people try more loci. We soon may be able to get the same titers that random integration gives.”
Lee brought the discussion back to the original question: “How many clones should we have going into the clinic, knowing that we’ll need one commercial cell line?” Panelists and attendees generally agreed that it’s not a common plan to make that change later on, but that sometimes it has to happen.
“If a legacy cell line was generated with older technology, and we can double productivity with a new one,” Sen explained, “then it’s worth going through the comparability studies and such for approvals to change at an appropriate phase. With a complex molecule, we might want a backup strategy.”
“We’ve had legacy products with different hosts,” Chakrabarti mentioned, “and started over with CHO.”
And Kumar said, “Ideally, if you can start with the best cell line by phase 1, then you want to stick with it throughout the life of the product. Some companies want to get to phase 1 very quickly and then switch the cell line (which can require a lot of work) once the product is successful in the clinic.”
“Preclinical companies don’t have time to optimize,” an attendee pointed out. “Sometimes you don’t have gene-sequencing data in time to make decisions, so you pick the two top-producing clones, for example. We’ve found sequence variants with our top clone before and had to go with the backup.”
Chakrabarti added, “I’ve seen issues with stability of cell lines (when it’s taken off the critical path).”
“If you can get quickly to the clinic, then you have to worry about comparability,” cautioned Kumar. He cited the case of a complex nonantibody protein originally expressed by a human cell line that didn’t offer high enough productivity, “so at a later phase [the developer] had to change to CHO for business needs.” In another case, product quality turned out to be a problem once detailed sequencing data were available. In a third case, a dramatic increase in productivity was needed for a very successful product, and the resulting cell-line change required comparability testing and even some clinical work. “In most cases that I’ve seen, changing cell lines was unplanned. Ideally you want to keep the same one all the way through.”
“We really don’t want to be switching cell lines,” Lee said, “and experience has shown us that the worst time to switch is postlicensing. But what about if you switch between clinical trials?”
It must be phase appropriate, said Sen. Material from two different cell lines would cause difficulties in getting regulatory approval. And Kumar added, “but if you have to do it then you have to do it.”
An audience member recounted having to make the change after phase 1, “and there were many hurdles. Now we try to keep the same cell line throughout. In licensing that can be important.” There’s less risk and regulatory burden, however, in making changes to an existing cell line than in switching to another one. Companies can “supertransfect” or reclone an existing cell line — e.g., to boost titers — with less risk of variability. And regulators seem to be more comfortable with that. But such decisions are made case by case. The attendee noted that expected demand and broadened indications can increase productivity needs. For a rare-disease treatment, titer is less important. But “you don’t always know what will arise later on. We want the best of both worlds — moving fast and getting one good cell line.”
So timelines drive risk, Lee said, which must be balanced by the amount of work that has to be done. But with speed-to-clinic/market as the primary driver, he asked, “Does the answer to the primary question change?” He pointed to pre- and post-COVID timelines and the increasing comfort with risk that may have resulted.
“That’s the new normal,” Chakrabarti said. Accelerating projects reduces the numbers of clones that can be screened by about half, she said, and “we haven’t seen an alarming increase of risk to product quality, stability, titer, and so on.”
Kumar added that the emergency situation provided the industry with an opportunity to learn how to do things quickly without compromising quality. Some companies now move into clinical trials with product from stable pools rather than cell lines. During COVID, one company received conditional approval with a nonclonal cell line. He also reminded the audience that before the FDA’s clonality emphasis began in 2014, biologics commonly went to phase 1 from minipools.
“COVID showed us that we can use stable-pool material to move very fast,” said Sen. “It’s a
calculated risk, and you use the pool to screen for your final cell line.”
“How is it different going from a pool to a single clone,” Lee asked, “rather than one clone to another?”
“If the pool is homogeneous,” Sen replied, “then you can justify some equality of product.”
Kumar pointed out that product quality from stable pools “in most cases will be very similar to that of a clone from that pool.”
Clonality has been more a regulatory emphasis than a scientific one. Clones change as they divide over time, anyway, which has lessened some of that strict emphasis since about 2018.
Kumar pointed out that one advantage for starting with targeted integration, however, may be that homogeneous beginning population relative to what random integration provides. “That may be part of why the anti-COVID antibody from pools was authorized.”
An attendee spoke from having developed a spike-protein vaccine. “Characterization has shown that material from both [pool and clone] is very similar. It’s a large, complex glycoprotein.” Someone else suggested that perhaps a single clone had taken over the population, which might explain the great similarity. But the pools were stable over at least 60 generations, and deriving clones from them takes a few weeks. The product from each of six independent pools and clones from them were very similar.
Kumar said that although minipools can turn out to be clonal when one clone takes over through time, bulk pools don’t tend to do the same.
References
1 Lietz CB, et al. Identification of Tyrosine Sulfation in the Variable Region of a Bispecific Antibody and Its Effect on Stability and Biological Activity. mAbs 15(1) 2023: 2259289; https://doi.org/10.1080/19420862.2023.2259289.
2 Yamano-Adachi N, et al. Establishment of Fast-Growing Serum-Free Immortalised Cells from Chinese Hamster Lung Tissues for Biopharmaceutical Production. Sci. Rep. 10(1) 2020: 17612; https://doi.org/10.1038/s41598-020-74735-0.
Cheryl Scott is cofounder and editor in chief of BioProcess International (part of Informa Connect Life Sciences); 1-212-600-3429; [email protected].