As described in ICH Q5A on virus safety of biotechnological products (1) and the European guideline on virus safety of biotechnological products, EMEA 398498 (2), viral clearance studies are mandated as part of the viral safety evaluation of products derived from human or other mammalian cell lines. When acceptable ranges of process parameters are known, both guidelines recommend that scale-down models be evaluated under worst-case conditions for viral clearance.
The BioPhorum Development Group’s viral clearance workstream performed a benchmarking survey to identify operating parameters that commonly are considered to present worst-case conditions for viral clearance. The survey further assessed whether companies evaluated process parameters differently for clinical trial stages (investigational new drug applications, INDs) and for commercialization (biologics license applications, BLAs).
Worst-Case Conditions
Worst-case conditions for a viral clearance step differ depending on its stage in a manufacturing process. In many cases, such conditions are process specific. A case-by-case assessment using process knowledge and current literature (where available) should be carried out for each method when determining worst-case conditions for any process. The information shared herein is not meant to provide an exhaustive list or offer specific recommendations, but to show what parameters are studied and controlled most frequently at worst case for several common viral clearance steps. The survey also shows which parameters are not controlled for worst-case studies.
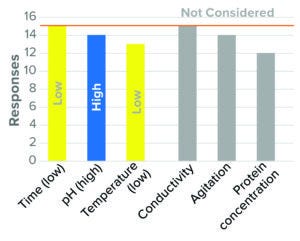
Figure 1: Virus inactivation, pH (15 respondents, BLA responses only).
Beginning with Figure 1 and running throughout the article, qualitative values (e.g., high, low) are used to indicate worst-case directionality for parameters considered by the majority of surveyed respondents. Gray bars indicate the level of response for parameters not considered in worst-case studies. Parameters not listed were those found to have a weak (<50%) assessment of directionality.
Table 1 lists the operations assessed in our survey and the general understanding of each step’s viral clearance mechanism.
Method of Analysis
Sixteen companies took part in the survey. The number of responses per question varied based on relevance to a company’s experience. Summarized survey responses focus on process steps in which ≥5 companies responded. A ≥50% response rate was used to eliminate process parameters that were not generally expected to compromise virus clearance or when directionality was not indicated clearly across the responses for a process step. Because the number of responses was variable, trends for some process steps were based on a small number of responses.
The bar graphs show results related to each process step. Each parameter is indicated by a color-coded bar. For response rates <100%, responses differed in directionality, level of consideration, or number of responses. In Figure 1, for example, 13 of the 15 responses considered low temperature to be a worst-case condition (as indicated by the yellow bar). The remaining three considered high temperature to be a worst-case parameter, did not consider the parameter, or did not respond. For protein concentration, 12 of 15 responses did not consider that condition as one compromising virus inactivation (as indicated by the gray bar). The other four respondents considered high or low protein concentration as a worst-case parameter or did not respond. The full list of survey responses is included in a “supporting information” file alongside the archived version of this article.
Virus Inactivation
Virus inactivation methods included in the survey were low-pH incubation, solvent–detergent (SD) incubation, and UV exposure. Of the 16 companies that participated in the survey, only two responded to questions on UV inactivation, so UV exposure is not discussed herein.
Low-pH Inactivation: Low-pH inactivation is a dedicated viral clearance step that reduces infectivity while maintaining a target molecule’s therapeutic activity. Cross-industry groups, including ours, have published data showing robustness of the step within specific conditions (3). ASTM International and industry publications have established key parameter ranges based on statistical evaluations of historical data (4, 5).
The results indicate that the majority of survey participants (≥87%) considered minimum inactivation time, high inactivation pH, and low temperature as worst-case conditions (Figure 1). Similar approaches were used for IND and BLA studies, with slightly fewer companies identifying temperature as a worst-case parameter at the IND stage. That result is consistent with scientific understanding that high pH and low temperature are least effective at disrupting a viral lipid envelope. Minimal time is understood to present a worst-case condition in terms of inactivation kinetics.
Most survey respondents did not consider conductivity, protein concentration, or agitation as having worst-case directionality. Although the effects of buffer type and strength are discussed in literature, conductivity has not been identified specifically as a critical parameter for pH inactivation (5, 6). Both high and low protein concentration were considered as worst-case parameters but with no clear consensus on directionality (7–9).
Solvent/Detergent Inactivation: The objective of SD and detergent-only treatments is to reduce the ability of enveloped viruses to bind and infect cells through disruption of their lipid envelopes. The survey did not distinguish between SD and detergent only.
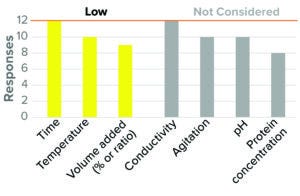
Figure 2: Virus inactivation, solvent/detergent (12 respondents, BLA responses only).
Most companies surveyed (≥75%) considered low inactivation time, low temperature, and low SD concentration (referred to in the survey as “SD volume added by % or ratio [of the starting protein solution]”) as worst-case conditions in BLA studies (Figure 2). These results reflect the common understanding of the mechanism of this step (10, 11). Similar trends were observed for IND studies.
Most respondents did not consider protein concentration to be a critical parameter for viral inactivation, although one-third of participants considered high protein concentration to be a worst-case condition. Current literature indicates that the influence of protein concentration on SD inactivation may be varied (12–14). The majority of participants (>80%) did not consider conductivity, agitation rate, and pH to have worst-case directionality.
Worst-Case Conditions for Chromatography Steps
Protein A Affinity Chromatography: Protein A chromatography is used widely as an initial capture step in protein purification. This step captures the protein in bind–elute mode through interaction of the molecule’s fragment crystallizable (Fc) region with a natural or engineered protein A ligand. Protein A chromatography is generally thought to be reproducible but less effective than dedicated viral clearance steps (13–15). The mechanisms of virus removal by protein A are more variable than other chromatography steps and hence the capture step might not perform consistently in individual processes (15–17).
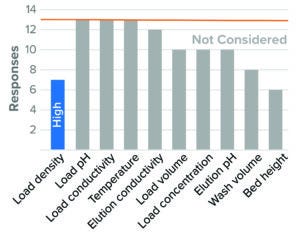
Figure 3: Affinity chromatography, protein A (13 respondents).
Figure 3 lists survey results for protein A affinity chromatography. Overall, identification of worst-case conditions was varied, and no parameter received an overwhelming response. This result is consistent with reported protein A chromatography studies that demonstrated how viral clearance levels remained consistent when several run parameters were varied (16, 17).
Resin load density was the one parameter that respondents considered to have an impact on virus clearance, with high load density presenting a worst-case directionality in BLA studies. Not indicated in the graph, a number of respondents also identified wide collection criteria and low wash volume as presenting worst-case cases (46% and 38% of total respondents, respectively).
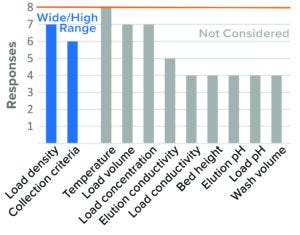
Figure 4: Bind–elute chromatography, cation-exchange (CEX) resin (8 respondents).
Other surveyed parameters were rarely or not considered. Those related to properties of the load material (pH, conductivity, and protein concentration), elution-buffer conductivity and pH, and temperature. Most respondents did not consider parameters relating to exposure of a feed stream to a resin (residence time, flow rate, and bed height). However, most respondents who did consider those parameters leaned toward longer exposure time as presenting a worst-case condition (long residence time, low flow rate, and high bed height). That may indicate a concern that nonspecific virus–resin interactions can compromise capacity for viral clearance. Conditions studied for BLA and IND filings were similar for all parameters in protein A affinity chromatography studies.
Bind–Elute Chromatography: Bind-and-elute chromatography often is used as a polishing step for monoclonal antibodies (MAbs) and other proteins. Across all bind–elute chromatography modes, high product loading is considered to be a worst-case parameter.
The bind–elute and membrane chromatography methods in the survey included anion exchange (AEX), cation exchange (CEX), hydrophobic interaction (HIC), mixed-mode AEX, and mixed-mode CEX. Out of the 16 companies, ≤3 companies responded to mixed-mode CEX, CEX membrane, and HIC membrane chromatography, so those are not discussed here. The full list of survey responses is included in the supporting information file.
CEX Bind–Elute Chromatography: CEX chromatography is an ion-exchange polishing chromatography step used for MAbs and other proteins. Published data indicate that CEX may be less effective for parvovirus clearance than for other model viruses such as murine leukemia virus (MuLV) and often can produce variable results. However, this mode can be robust under relatively low-pH buffer conditions (18, 19). The two parameters considered to be most critical to virus clearance by CEX were resin load density and collection criteria.
The majority of respondents (90%) considered high load density to be a worst-case condition. Having wide collection criteria for BLA studies was considered to be a worst-case condition by 80% of companies. Although IND trends were similar to those reported in BLA results, fewer respondents placed collection criteria under worst-case conditions in IND studies.
Other parameters were not considered by the majority of respondents, or no clear consensus emerged. High load density and wide collection criteria would increase the potential for impurities to bind and potentially coelute with product based on current literature (19).
AEX Resin and Membrane Bind–Elute Chromatography: AEX chromatography is commonly used as a polishing step for MAbs and other proteins. For both resin- and membrane-based chromatography, bind–elute AEX generally is understood to be a less-robust viral clearance step, so it is used less commonly than flow-through mode (20, 21).
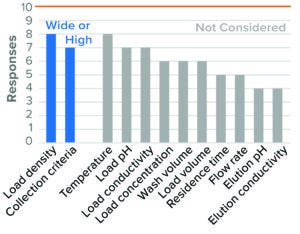
Figure 5: Bind–elute chromatography, anion-exchange (AEX) resin (10 respondents).
For BLA studies, the two parameters considered to be most critical to AEX were resin/membrane load density and collection criteria. Most survey respondents considered high load density and wide collection criteria as worst-case conditions (Figures 5 and 6). Although IND and BLA trends were similar, fewer respondents considered those parameters as worst-case conditions in IND studies. Other parameters were not considered by most of the respondents or showed no clear directionality.
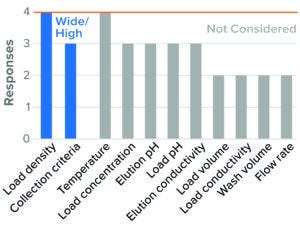
Figure 6: Bind–elute chromatography, AEX membrane (4 respondents).
HIC Bind–Elute Chromatography: HIC is used as a polishing chromatography step for MAbs and other proteins. The number of respondents for this mode (five for BLA studies and seven for IND studies) indicates that it is less likely to be relied upon for virus clearance than other chromatographic modes.
The parameters considered in evaluating worst-case conditions in BLA studies were high resin load density and wide collection criteria. Trends were similar for IND studies, but with fewer respondents (Figure 7). Those data are included in the supporting file.
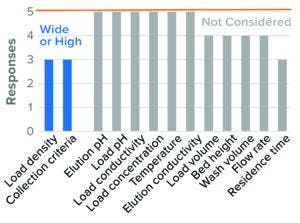
Figure 7: Bind–elute chromatography, HIC resin (5 respondents).
Flow-Through Chromatography: Flow-through chromatography is commonly used as a polishing step for MAbs and other proteins. Across all flow-through chromatography modes, high product loading is considered to be a worst-case parameter because it would maximize the possibility of viruses exceeding the resin binding capacity.
Flow-through resin and membrane chromatography methods included in the survey were AEX, CEX, HIC, mixed-mode AEX, and mixed-mode CEX. Out of the 16 companies, 14 of them responded to AEX; five responded to HIC and mixed-mode AEX each. The other steps drew fewer responses and were not discussed. The full list of survey responses is included in the supporting information file.
AEX Resin and Membrane Flow-Through Chromatographies: AEX resin flow-through chromatography is a commonly used polishing step for MAbs and other proteins. It is generally known to be a robust viral clearance step (21).
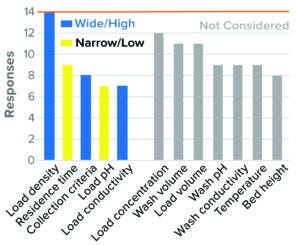
Figure 8: Flow-through chromatography, AEX resin (14 respondents).
The survey results indicate that for most participants, high load density, low residence time, wide flow-through collection criteria, low load pH, and high load conductivity were considered to present worst-case conditions in BLA studies (Figure 8). Similar approaches were used for IND studies as well but drew fewer respondents. This was the only purification step in which load parameters such as load pH and conductivity were considered to be worst-case conditions. Other parameters such as load concentration, load volume, wash pH, and conductivity and temperature were not considered by the majority of respondents.
Compared with the use of resin chromatography, fewer survey respondents indicated using AEX membrane chromatography for virus clearance. All four respondents studied high load density and wide flow-through collection criteria in worst-case BLA studies (data not shown).
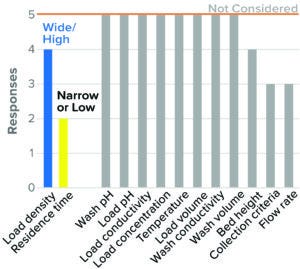
Figure 9: Flow-through chromatography, hydrophobic-interation (HIC) resin (5 respondents).
HIC Flow-Through Chromatography: HIC flow-through chromatography is used as a polishing chromatography step for MAbs and other proteins. The relatively low number of respondents (five) indicates that it is less likely to be used for viral clearance than other forms of chromatography. Most respondents studied high resin load density and low residence time as worst-case conditions in BLA studies (Figure 9). Other parameters such as load concentration, load volume, load pH and conductivity, wash pH, and conductivity and temperature were not considered by a majority of the respondents.
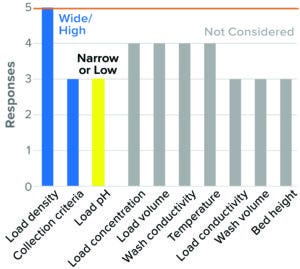
Figure 10: Flow-through chromatography, mixed-mode AEX (5 respondents).
Mixed-Mode AEX Flow-Through Chromatography: Use of mixed-mode AEX flow-through chromatography is relatively new to downstream processing. Although this part of the survey drew in relatively few responses, the body of information related to this step is expected to grow. High resin load density, wide flow-through collection criteria, and low load pH were considered to be worst-case conditions. Other parameters such as load conductivity, wash pH and conductivity, temperature, and residence time were not considered, or a consensus on directionality was not found (Figure 10).
Virus-Retentive Filtration
Virus-retentive filtration (VRF) is commonly used for removal of viruses in biopharmaceutical manufacturing. Cross-industry groups, including ours, have published data showing robustness of the step within specific conditions (22).
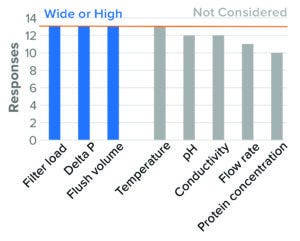
Figure 11: Virus-reduction filtration — BLA (based on 13 respondents).
Multiple VRF formats are available and can be operated in normal or tangential flow. Only three of the 16 surveyed companies reported using tangential-flow filtration (TFF), whereas all 16 companies indicated using normal-flow filtration. Parameters surveyed are shown in Figure 11. For tangential flow, the survey results are unclear because of the low response rate and lack of clarity about whether hollow-fiber filters were operated in tangential or normal-flow mode.
Normal Flow: For normal-flow operation, the parameter considered to be most critical to virus filtration was membrane load (volume or mass filtered per unit area). All companies surveyed identified high membrane load as a worst-case condition for both BLA and IND studies.
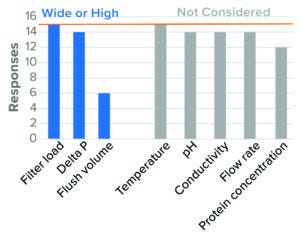
Figure 12: Virus-reduction filtration — IND (based on 15 respondents).
Differential pressure also was identified as a worst-case parameter, with clear worst-case directionality at both extremes (high and low). Literature suggests that high pressure presents a worst case because of the simple sieving effect of particles based on size. Other literature cites parvovirus breakthrough under conditions of low pressure and pressure interruption (23). For BLA studies, the majority of surveyed companies evaluated both high and low pressure. For IND studies, the majority of respondents evaluated only high pressure. Some regulatory agencies say that they expect a range of pressures to be tested (24). High-recovery flush volume was studied as a worst case in BLA studies, with fewer respondents considering that parameter during IND studies (Figure 12). Few companies considered product pH and conductivity, flow rate, and protein concentration. No companies considered temperature in worst-case studies.
The survey also addressed certain details of executing virus filtration studies such as viral-load control methods, methods for driving flow, and pressure/flow interruptions. Virus spike volume (%) was most commonly evaluated by respondents to control viral load, closely followed by total virus challenge (in logs). Target LRV was rarely used by the companies surveyed. The majority use air-pressure–driven flow to perform small-scale viral filtration studies.
An industry-wide concern is that small viruses can pass through VRFs. This phenomenon is widely reported and has been demonstrated under conditions of low pressure and pressure interruption. The survey revealed that most companies tend to evaluate the impact of pressure interruptions as part of their best practice. For BLA studies, the majority of companies evaluated one pressure interruption, whereas some evaluated two interruptions. Most companies include interruptions at the end of load before flush (11 out of 16 respondents), whereas some companies considered additional interruptions at middle/end of loading (two of 16), at start/end of loading (one of 16), and at start/middle of loading (one of 16). Although not specifically surveyed here, it is recommended in the literature that companies evaluate depressurization events when designing VRF studies in relation to their manufacturing processes (25–27).
Literature contains no reports of protein concentration affecting viral clearance, although buffer pH and conductivity do influence small-virus passage (22, 23, 28, 29).
Process Parameters for Virus Activation and Removal
This benchmarking survey identified process parameters that commonly are selected for studying worst-case conditions for virus inactivation and virus removal steps. The survey also established the directionality of those worst-case parameters in a virus clearance study. Outcomes of the survey were discussed related to steps that received a significant number of responses: virus inactivation (low pH and SD), protein A affinity chromatography, bind–elute chromatography (AEX, CEX, HIC, mixed-mode AEX, and mixed-mode CEX), flow-through chromatography (AEX, HIC, mixed-mode AEX) and virus reduction filtration. The number of responses was variable, and for some process steps the trend was drawn from only a few respondents.
The survey results revealed a strong consensus about how to approach studies for virus inactivation steps. Low temperature, minimum incubation time, and high pH were considered to present worst-case conditions for low pH inactivation. Low temperature, minimum incubation time, and low SD concentration were considered to be worst-case conditions for low pH and SD inactivation, respectively.
Resin load density and collection criteria were parameters selected by the majority of companies for worst-case studies of both bind–elute and flow-through chromatography steps. Other parameters such as operating temperature, protein concentration, and wash conditions were not prioritized. In AEX and multimodal AEX flow-through chromatography, parameters related to load properties (e.g., load pH and conductivity) and parameters related to column-product exposure (e.g., residence time) also were considered by a significant number of companies.
For VRF, high filter load and differential pressure (high and low) were the most significant factors across all companies surveyed. Respondents also considered including pressure interruptions and a high recovery flush volume as best practices for such studies.
The survey indicated minimal differences in the ways that companies approach BLA and IND studies. In cases where a different approach was taken, BLA studies tended to be more conservative, in that more parameters were tested under worst-case conditions.
References
1 CPMP/ICH/295/95. ICH Q5A (Step 4, Consensus Guideline): Quality of Biotechnological Products — Viral Safety Evaluation of Biotechnology Products Derived from Cell Lines of Human or Animal Origin. International Council for the Harmonisation of Technical Requirements for Pharmaceuticals for Human Use: Geneva, Switzerland, 4 March 1997; http://www.pharma.gally.ch/ich/q5a029595en.pdf.
2 EMEA/CHMP/BWP/398498/2005. Virus Safety Evaluation of Biotechnological Investigational Medicinal Products. European Medicines Agency: Amsterdam, Netherlands, 2009; https://www.ema.europa.eu/en/documents/scientific-guideline/guideline-virus-safety-evaluation-biotechnological-investigational-medicinal-products_en.pdf.
3 Matilla J, et al. Retrospective Evaluation of Low-pH Viral Inactivation and Viral Filtration Data from a Multiple Company Collaboration. PDA. J. Pharm. Sci. Technol. 70(3), 2016: 293–299; https://doi.org/10.5731/pdajpst.2016.006478.
4 Standard Practice for Process for Inactivation of Rodent Retrovirus by pH. ASTM Standard E2888. ASTM International: Washington, DC, 2012.
5 Brorson K, et al. Bracketed Generic Inactivation of Rodent Retroviruses by Low pH Treatment for Monoclonal Antibodies and Recombinant Proteins. Biotechnol. Bioeng. 82(3) 2003: 321–329; https://doi.org/10.1002/bit.10574.
6 Durno L, Tounekti O. Viral Inactivation: Low pH and Detergent. PDA J. Pharm. Sci. Technol. 69(1) 2015; 163–172; https://doi.org/10.5731/pdajpst.2015.01040.
7 Chinniah S, Hinckley P, Connell-Crowley L. Characterization of Operating Parameters for XMuLV Inactivation by Low pH Treatment. Biotechnol. Prog. 32(1) 2016: 89–97; https://doi.org/10.1002/btpr.2183.
8 Chen Q. Viral Clearance Using Traditional, Well-Understood Unit Operations (Session 1): Low-pH Inactivation. PDA J. Pharm. Sci. Technol. 68(1) 2014: 17–22; https://doi.org/10.5731/pdajpst.2014.00962.
9 Kreil T, Roush, D. Proceedings of the 2017 Viral Clearance Symposium, Session 2.1: DSP Unit Operations — Virus Filtration/Inactivation. PDA J. Pharm. Sci. Technol. 72(5) 2018: 470–478; https://doi.org/10.5731/pdajpst.2018.009118.
10 Standard Practice for Process Step to Inactivate Rodent Retrovirus with Triton X-100 Treatment. ASTM E3042-16, ASTM International 2016; https://standards.globalspec.com/std/10043236/astm-e3042.
11 Brorson K, et al. Conference Summary: Gaps, Lessons Learned, and Areas for Improvement. PDA J. Pharm Sci and Technol. 68(1) 2014: 83–89; https://doi.org/10.5731/pdajpst.2014.00968.
12 Guidelines on Viral Inactivation and Removal Procedures Intended to Assure the Viral Safety of Human Blood Plasma Products (Annex 4, TRS 924). World Health Organization: Geneva, Switzerland, 2004; https://www.who.int/publications/m/item/WHO-TRS924-Annex4.
13 Miesagaes, G. et al. Proceedings of the 2009 Viral Clearance Symposium. Dev. Biol. (Basel). 133, 2010: 3–101; PMID: 21516942.
14 Roberts, P. Virus Inactivation By Solvent/Detergent Treatment Using Triton X-100 in a High Purity Factor VIII. Biologicals. 36(5) 2008: 330–335; https://doi.org/10.1016/j.biologicals.2008.06.002.
15 Bach J, Connell-Crowley L. Clearance of the Rodent Retrovirus, XMuLV, By Protein A Chromatography. Biotechnol. Bioeng. 112(4) 2015: 743–745; https://doi.org/10.1002/bit.2548.
16 Zhang, M, et al. A Novel Q-PCR Based Approach to Measuring Endogenous Retrovirus Clearance By Capture Protein A Chromatography. Biotechnol. Bioeng. 102(5) 2009: 1438–1447; https://doi.org/10.1002/bit.22172.
17 Zhang M, et al. Quality By Design Approach for Viral Clearance By Protein A Chromatography. Biotechnol. Bioeng. 111(1) 2014: 95–103; https://doi.org/10.1002/bit.24999.
18 Miesagaes G, et al. Monoclonal Antibody Capture and Viral Clearance by Cation Exchange Chromatography. Biotechnol. Bioeng. 109(8) 2012; 2048–2058; https://doi.org/10.1002/bit.24480.
19 Connell-Crowley L, et al. Cation Exchange Chromatography Provides Effective Retrovirus Clearance for Antibody Purification Processes. Biotechnol. Bioeng. 109(1) 2012: 157–165; https://doi.org/10.1002/bit.23300.
20 Cipriano D, et al. Effectiveness of Various Processing Steps for Viral Clearance of Therapeutic Proteins: Database Analyses of Commonly Used Steps. Methods in Molecular Biology 899. Therapeutic Proteins: Methods and Protocols. Voynov V, Caravella JA, Eds. Springer Nature: London, UK, 2021: 277–292.
21 Remington K, et al. Viral Clearance By Protein A, Anion Exchange and Cation Exchange Chromatography Steps. Am. Pharm. Rev. 30 November 2015; https://www.americanpharmaceuticalreview.com/Featured-Articles/181836-Viral-Clearance-by-Protein-A-Anion-Exchange-and-Cation-Exchange-Chromatography-Steps.
22 Mattila J, et al. Retrospective Evaluation of Low-pH Viral Inactivation and Viral Filtration Data from a Multiple Company Collaboration. PDA. J. Pharm. Sci. Technol. 70(2) 2016: 293–299; https://doi.org/10.5731/pdajpst.2016.006478.
23 Dishari K, et al. Effects of Solution Conditions on Virus Retention By the Viresolve® NFP Filter. Am. Inst. Chem. Eng. Biotechnol. Prog. 31(5) 2015:1280–1286; https://doi.org/10.1002/btpr.2125.
24 Kreil TR, Roush D. DSP Unit Operations – Virus Filtration/Inactivation. Session 2.1, Proceeding of the 2017 Viral Clearance Symposium. PDA. J. Pharm. Sci. Technol. 72(5) 2018: 470–478; https://doi.org/10.5731/pdajpst.2018.009118.
25 Strauss D, et al. Characterizing the Impact of Pressure on Virus Filtration Processes and Establishing Design Spaces to Ensure Effective Parvovirus Removal. Biotechnol. Prog. 33(5) 2017: 1294–1302; https://doi.org/10.1002/btpr.2506.
26 Chen Q, Chen D. Viral Clearance of Traditional Unit Operations: Virus-Retentive Filtration. PDA. J. Pharm. Sci. Technol. 69(1) 2015: 142–153; https://doi.org/10.5731/pdajpst.2015.01038.
27 Dan LaCasse, et al. Impact of Process Interruption on Virus Retention of Small-Virus Filters. BioProcess Int. 11(10) 2013: 34–44; https://bioprocessintl.com/upstream-processing/biochemicals-raw-materials/impact-of-process-interruption-on-virus-retention-of-small-virus-filters-347992.
28 Marques BF, Roush DJ, Göklen KE. Virus Filtration of High-Concentration Monoclonal Antibody Solutions. Biotechnol. Prog. 25(2) 2009): 483–491; https://doi.org/10.1002/btpr.177.
29 Stuckey J, et al. A Novel Approach to Achieving Modular Retrovirus Clearance for a Parvovirus Filter. Biotechnol. Prog. 30(1) 2014: 79–85; https://doi.org/10.1002/btpr.1820.
Dhiral A. Shah is a senior scientist at Sanofi in Framingham, MA. James Berrie is a technical director at Lonza in Basel, Switzerland. Jeremy Pike is associate director of downstream process development at Alexion Pharmaceuticals, Inc. in Boston, MA. Susan M. Liu is with Janssen R&D in Malvern, PA. Sherrie Curtis is principal scientist at Roche/Genentech Inc. in South San Francisco, CA. David Roush is a distinguished scientist in biologics PR&D at Merck & Co., Inc. in Kenilworth, NJ, USA. Nuria DeMas is associate director and senior technical steward in biologics manufacturing operations at Novartis (and is a contributing editor to this article). Atul Bhangale is a senior principal scientist and represents the views of Bristol-Myers Squibb, Devens MA, USA. Corresponding author Nadine Hazelwood ([email protected]) is the client delivery director, and Justin John is a facilitator at BioPhorum.
Supporting information from the survey results can be found alongside this article on the BPI website at https://bioprocessintl.com/featured-report/november-december-2022-featured-report-viral-safety-focus-on-inactivation-and-clearance.