Buffers are used widely in molecular biology, biochemistry, and biopharmaceutical manufacturing. For the latter, buffers play a pivotal role in modulating the characteristics of biologics, influencing their interactions with other molecules and surfaces as well as the structure and stability of the biologics themselves (1). Biopharmaceuticals invariably are formulated with buffers, which serve to stabilize them and affect their physiological transport and function. Buffers are used extensively in biopharmaceutical processes, particularly chromatography steps in downstream processing (2). A buffer’s pH level can affect significantly the state of a chromatographic resin and protein of interest, determining the effectiveness of binding and elution of both products and impurities (3).
To design buffers effectively, we must understand basics of their chemistry and reactions, including how those relate to solution pH and ionic concentrations. Once buffers are designed, we can analyze them for compound choice and concentration, ion concentrations, and variability. Here we explore those aspects while highlighting the use of a free online tool that we recently launched: the “Expert Buffer Designer” at https://bioprocess-tools.app.
Designing Buffers
Phosphate, acetate, citrate, tris, and histidine are some of the most prevalent buffer families (species) used in biopharmaceutical processes. Here we use the Expert Buffer Designer to design and understand buffers step by step according to a typical workflow (Figure 1).
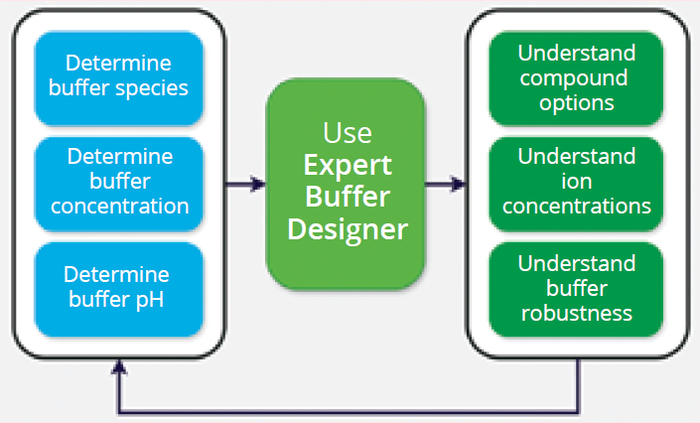
Figure 1: Buffer design workflow.
Step 1: Selecting buffering species starts with determining the desired pH range for a given process step. For instance, during monoclonal antibody (mAb) purification, an acetate or citrate buffer might be optimal for antibody binding to cation-exchange (CEX) resin, whereas tris or phosphate might be optimal for affinity chromatography. Although pH is a crucial factor, we also must consider the charge of a buffering species and its effect on resin chemistry. The multivalency of phosphate and citrate, for example, can hinder anion-exchange (AEX) flowthrough performance (4).
Understanding the dissociation reactions of buffering species provides insights into charge characteristics, effective pH ranges, and complexities in the interplay between pH and ion concentrations. Each buffer species undergoes ionization equilibrium reactions, sequentially dissociating hydrogen ions at characteristic dissociation constants (pKa) (5, 6). Phosphate, for example, undergoes three dissociations, each with a corresponding pKa, so it is a fitting buffer for maintaining pH ~7.2 (Equation 1).

Equation 1: Phosphate ionization reactions.
Choosing a buffer species is straightforward with the Expert Buffer Designer tool. Begin typing the first few letters into the autocomplete input box, and the tool will suggest relevant options (Figure 2). The tool contains an extensive library of buffer species used in bioprocessing, standard biochemical reactions, and Good’s buffers and a comprehensive collection of dual-species buffers. That makes the tool fast and convenient for many applications (7).
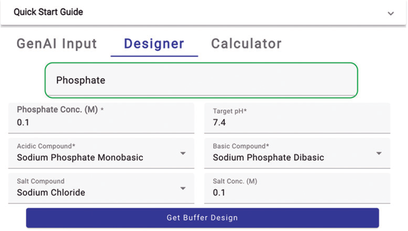
Figure 2: Buffer species selection (screenshot from the Expert Buffer Designer tool).
Step 2: Selecting buffer compounds has significant implications for both performance and operational efficiency. Typically, you can design a buffer by combining a species-containing compound with another (nonbuffering) compound that provides counterions to adjust the pH level. In designing a phosphate buffer, for example, one option is to use sodium phosphate monobasic (NaH2PO4) and sodium hydroxide (NaOH), with sodium serving as the counterion to shift pH to a desired level.
Alternatively, you can choose compounds that both contain your buffering species (Figure 3a), such as when combining the monobasic NaH2PO4 with sodium phosphate dibasic (Na2HPO4). This approach is rooted in classic biochemistry/molecular biology protocols, in which scientists typically combine stock solutions of those compounds proportionately to achieve a target pH.
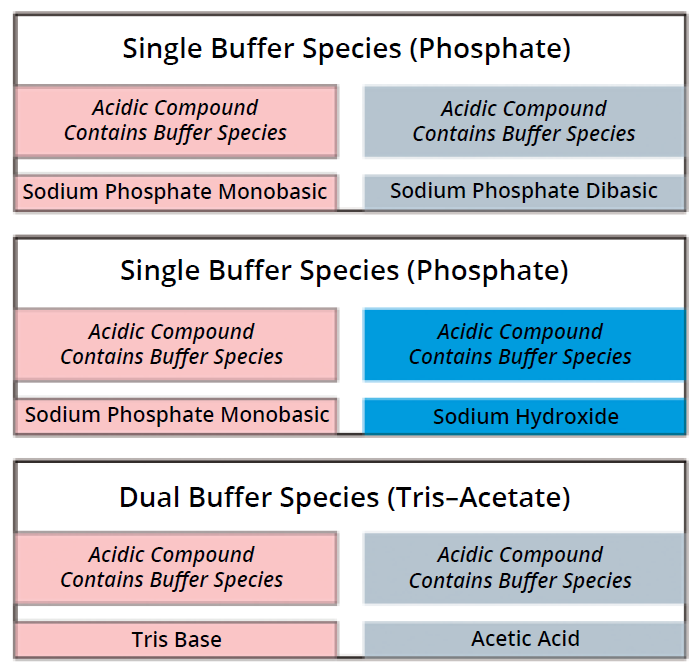
Figure 3a: Compound options.
When compounds dissolve in water, they dissociate into ions (Figure 3b). As such, you can use compounds such that ions in the solution remain essentially the same. Thus, we can design buffers with the same pH and composition based on either compound combination within a practical use range. However, consider operational aspects as well: e.g., the cost of raw materials, testing, and release processes; safe handling of chemicals; and factors such as solubility, hydration levels, and effects of storage, shipping, and handling.
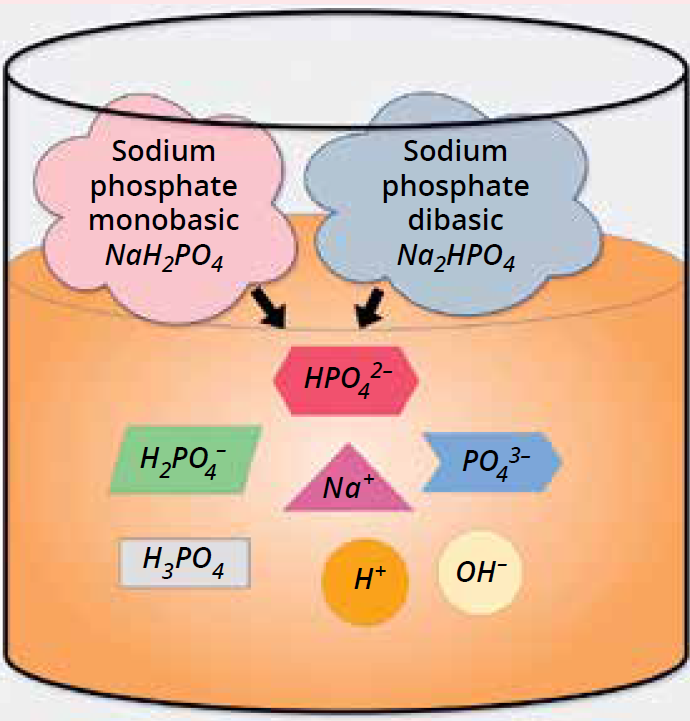
Figure 3b: Compound ionization.
Our Expert Buffer Designer tool simplifies compound selection. The tool intelligently presents only the compound choices that can be used to prepare a specified buffer to the target concentration. You can choose from a simple acid or base, a compound with the same buffer species, or a different buffer species that could act as a counterion (Figure 4).
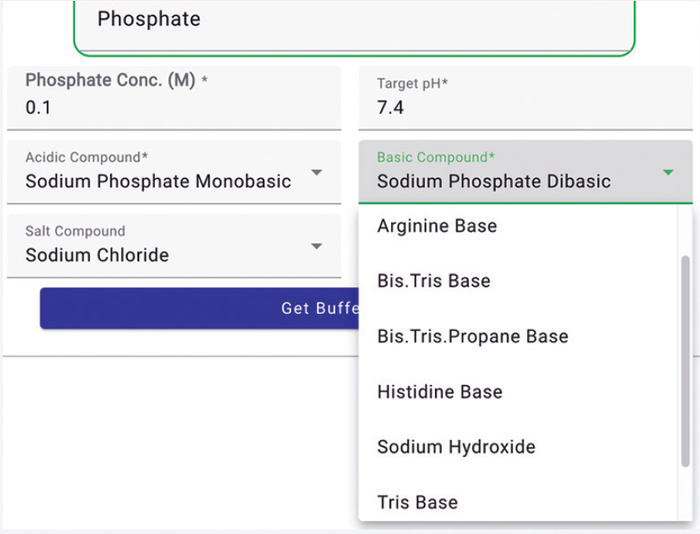
Figure 4: Selecting compounds from options (screenshot).
Step 3: Determining the buffer concentration can be iterative. It requires understanding how pH and ion concentrations influence a solution’s buffering capacity, buffer-ion charges, and ionic interactions with proteins and resins.
As Equation 1 shows, phosphate ions exhibit different degrees of dissociation in a solution based on the pH. You can manipulate that — and consequently, the degree of dissociation — by adjusting the counterion concentration while keeping the buffer species concentration constant. (Buffer concentration describes the total concentration of all buffer ions in a solution.) From the dissociation reactions and their equilibria, we determine individual species concentrations as a function of the total ionic concentration and pH (Equations 2–7).
Equations 2–7: Ionic concentrations and pKa adjustment.
Next, we incorporate a fundamental rule dictating the relative proportions of ions (including hydrogen ions) and the electroneutrality principle. Equation 8 represents that principle for the phosphate buffer system. Qualitatively, the relative level of sodium ions dictates the charge balance, which then influences the phosphate-ion dissociation, subsequently affecting the hydrogen-ion concentration and thus the solution pH.

Equation 8: Electroneutrality equation.
When deciding on buffer concentration and pH, you need to pinpoint those charged-ion concentrations and the pH level that will yield your desired ion distribution. Our tool streamlines those considerations: You can input different buffer concentrations and pH values, and it will display the ions’ relative proportions to help you determine the optimal buffer. If your requirements are more straightforward, you might choose a concentration based on best practices and a pH required for your specific process step. You also have the option to introduce salt into a buffer by selecting a suitable salt and its concentration. The online tool also corrects pKa based on the solution’s ionic strength using a well-established approach and iterative calculation (6). Once you have chosen all necessary inputs, click on the Design Buffer button to trigger the design calculations, the output of which will be returned to the user interface.
Buffer Design with Natural Language Input: Large language models (LLMs) make it possible to transform natural-language input from users into function inputs for application programming interfaces (APIs). We have leveraged the ChatGPT API (gpt-3.5-turbo-0125) to develop an algorithm that seamlessly enables the capability in our Expert Buffer Designer tool. A straightforward command such as “50-mM phosphate buffer, pH 7.4” returns a recipe equivalent to one derived from the multiple-input buffer designer interface featured in the subsequent tab (Figure 5). You can specify that you want to use sodium hydroxide as a counterion compound by requesting “50-mM Phosphate–NaOH buffer, pH 7.4” instead.
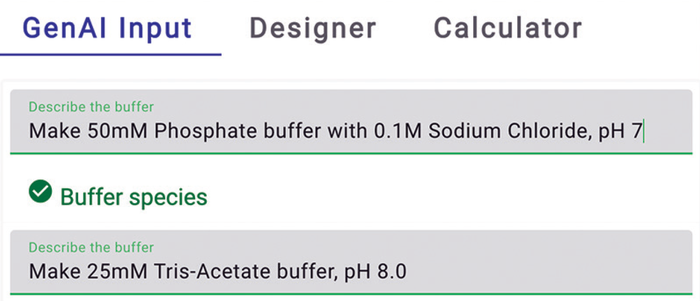
Figure 5: Natural language input (screenshot).
Behind the scenes, the tool interfaces with the ChatGPT API in real-time. Using a wealth of training data and prompts, along with its own proficiency in buffers and compounds, the tool translates user queries into a structured format. Our API then parses the output and invokes the necessary functions to generate a buffer recipe.
pH Calculation Sandbox: The Expert Buffer Designer toolbox includes a pH calculator, which gives you a testing ground for buffer development. It lets you freely select and combine chemicals, explore resulting pH levels, and understand the resulting compositions and their potential variations. The capability thus enables you to design unconventional buffers and understand extreme ranges of variability.
This flexible feature is not just for complex buffer systems; it’s also adept at calculating pH for single-component solutions in concentrations at which the Debye–Hückel corrections apply. Figure 6 shows the interface where you can input variables and instantly receive both the pH and the associated recipe. That direct feedback loop makes the calculator an indispensable asset for buffer designers seeking to push the boundaries of buffer science.
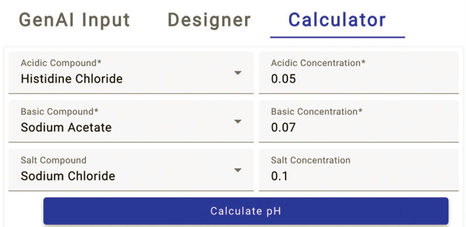
Figure 6: pH calculator input (screenshot).
Methodology Behind pH Calculations: We’ve established a workflow for calculating pH (Figure 7). Our tool takes the buffer concentration, chosen compounds, and target pH as inputs, then calculates the compound concentrations that will provide the target buffer species concentration while achieving a target pH level. In the phosphate buffer example, the tool determines molar concentrations of sodium phosphate monobasic and dibasic required to achieve the desired concentration, considering stoichiometry and other factors. The ionic strength is calculated and used to adjust pKa iteratively. When a target pH is achieved, the compound and ion concentrations are created and a heat map is provided to the user interface (Figure 8a).
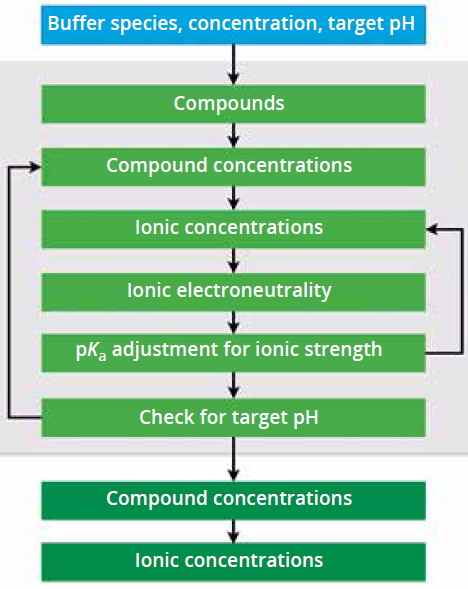
Figure 7: Calculation methodology.
As evident herein, the calculations are intricate, involving the resolution of highly nonlinear equations spanning 14 orders of magnitude. Through years of dedicated development, we’ve honed our algorithm to deliver speed, accuracy, and robustness — all without relying on complex software packages. This advancement is pivotal in making the Expert Buffer Designer tool freely accessible online, enabling its democratization and global adoption.
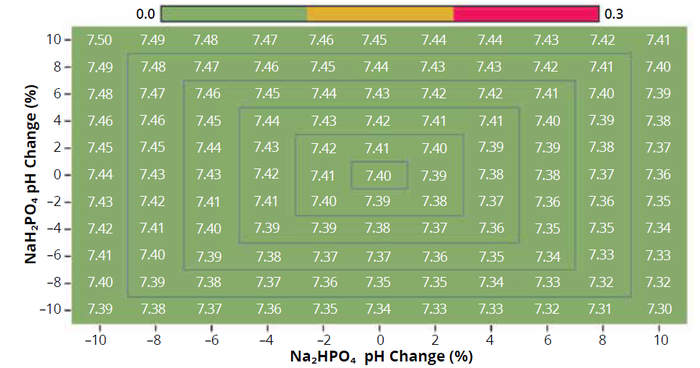
Figure 8a: Compound variability heatmap showing slight pH level changes.
Output: Insights and Optimization
Regardless of the input method used — generative AI input, the Expert Buffer Designer interface, or the pH calculator — the online tool always provides an updated buffer list, a buffer-recipe summary, and a detailed view containing compound concentrations, ion concentrations, and a compositional variability analysis heat map. This consistency ensures that users have the necessary data to fine-tune their own buffer formulations. The tool also allows you to edit outputs seamlessly using the designer or calculator.
Figure 8b shows a summarized output of the pH calculation and buffer list. The tool provides a convenient way to build an optimized list of buffers you can print and analyze.
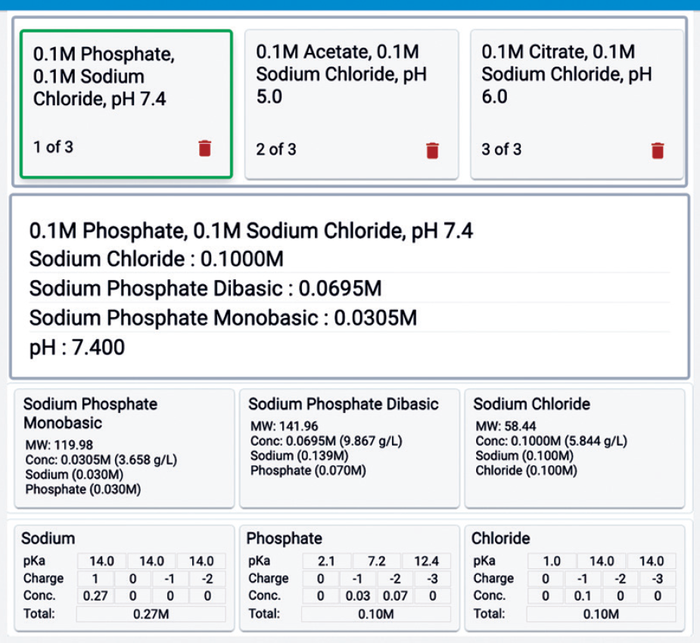
Figure 8b: Buffer list, summary, and details (screenshot).
Refining Compound Concentrations for Optimal Buffers: Compound choices can vary for specific buffer species. You can experiment with different combinations and assess their effects on compound concentrations by viewing compound molarities in the summary recipe and concentrations in the compound details (Figure 8b).
Ion Concentrations: We’ve established how critical ion concentrations influence protein and resin interactions. Once compounds dissolve, ions shed their compound identity, merging into a unified pool. The Expert Buffer Designer tool provides an exhaustive view of all compounds and ions (Figure 8b).
Understanding unique ion concentrations is critical to anticipating how buffers will interact with resins. If you observe, for instance, that the total concentration of negative ions (chloride and phosphate) is 0.2 M, and the total positive ion concentration (sodium) stands at 0.27 M, then you can infer the potential effects on binding interactions in CEX and AEX columns.
Additionally, the online tool provides a concentration of each ionic form. With that information, you can gauge the total charged-ion concentration in a given solution, which carries significant implications for ion-exchange chromatography and other applications in which charge interactions are critical.
Buffer Performance and Variability
One problem we face as buffer designers is predicting buffer robustness in practical scenarios. Since we cannot design buffers exclusively at their pKa, we must anticipate how pH might fluctuate due to inevitable variations during preparation and use. During use, those might include a resin’s adsorption or desorption of ions and the dynamic processes of protein binding and elution, which subsequently alters both pH and ion composition.
Preparation-related variability is critical to establishing an appropriate control strategy, taking into account variability in weighing and dispensing and volume additions due to the precision and accuracy limitations of measurement systems. Furthermore, when buffers are not made from preestablished recipes but rather prepared by titration, we encounter additional variabilities, such as the precision of acid/base addition, the pH sensor’s reliability, and the calibration of pH meters.
Traditionally, “buffering capacity”— the pH change resulting from adding a unit amount of acid or base — has been the standard metric for buffer characterization. However, that measure doesn’t help you understand variabilities. We advocate for a pragmatic solution that models variability as fluctuations in compound concentrations. Our Expert Buffer Designer tool, with its capacity for rapid and precise pH calculations, is engineered to vary compound concentrations over a range and display the resulting pH values on a heat map.
The heat map in Figure 8a for a phosphate buffer reveals the pH impact of a 30% variability in compound concentration. By setting visualization thresholds at 0.1, 0.2, and >0.3 fractions of pH, we observe that the variability is not uniform across the board. That insight is important because it shows that pH might be more sensitive to fluctuations in one compound than it is in another. This counters the common but erroneous belief that achieving a target pH implies compositional accuracy — a fallacy that often is encountered with titration methods, in which the correct pH might be reached only at the cost of compositional integrity.
Leveraging such insights through in silico methods vastly enhances the consistency and performance of the resulting buffers. Our approach exhibits operational robustness and embeds an effective control strategy into the buffer-design process. We champion this approach as an efficient quality-by-design (QbD) practice (8). In silico models enable such design for quality, removing the traditional reliance on characterization, verification, and validation.
Exploring Dual-Species Buffers — Insights from Tris–Acetate Systems: Dual-species buffers are well established in biochemistry and molecular biology. Their application in bioprocesses has been limited, however, because of complexity and limited understanding about their potential advantages. Studies demonstrate that dual-species systems such as the tris–acetate system have significant value in process simplification (9).
Buffer systems typically are selected to provide effective buffering around their pKa values. However, achieving robust pH control is paramount in processes that require precise pH adjustments (e.g., pH inactivation, neutralization, or sample preparation for CEX). An overshoot in pH, particularly at lower values (typically 3.5–3.7) necessary for pH inactivation, can damage proteins. In such cases, using an acetate buffer system with pH adjustments based on acetic acid, proves to be advantageous. Acetic acid becomes less ionized as a target pH approaches, leading to a gradual pH change overall. Conversely, tris exhibits similar behavior when pH is adjusted upwards and becomes less ionized near pH 8.0, ensuring robust control. That allows for rapid changes during transitions, enabling efficient attainment of target pH levels.
The Expert Buffer Designer tool makes designing such dual-species buffers straightforward. The designer interface presents a comprehensive list of potential pairings for specified buffer species, enabling creation and evaluation of several combinations under different process scenarios. That capability helps users determine the most effective system for their needs. We are excited to announce that we are working on modeling titrations in the next major version of this tool.
Filling an Industry Need
We’ve explored the intricacies of buffer design in the context of our Expert Buffer Designer online tool. Our design journey began with selecting buffer species and compounds, highlighting how those choices affect the overall effectiveness and operational feasibility of the resulting buffers. Herein we demonstrate the value and use of natural language inputs to simplify the input process and a pH calculator for broad exploration.
Through meticulous calculation of pH and compound concentrations, the tool can handle different input methods consistently, whether through AI-driven interfaces or traditional pH calculators. Buffer robustness is determined through simulations.
This represents a unique effort to provide a comprehensive tool for buffer design, which we saw as an unmet need in the biopharmaceutical industry. Making such a tool freely accessible through the online interface is another step in our vision of democratizing technology to help accelerate the development of essential patient treatments.
References
1 Strickley RG, Lambert WJ. A Review of Formulations of Commercially Available Antibodies. J. Pharmaceut. Sci. 110(7) 2021: 2590–2608.e56; https://doi.org/10.1016/j.xphs.2021.03.017.
2 Linderholm A, et al. Buffers in Biologics Manufacturing. BioProcess Int. 15(2) 2017: 20–27; https://www.bioprocessintl.com/qa-qc/buffers-in-biologics-manufacturing.
3 Pabst TM, Carta G. pH Transitions in Cation Exchange Chromatographic Columns Containing Weak Acid Groups. J. Chromatogr. A 1142(1) 2007: 19–31; https://doi.org/10.1016/j.chroma.2006.08.066.
4 Fuchs T, Jupke A. Comparison of the Impact of Anion and Cation Selection onto Cation Exchange Chromatography of Model Proteins. J. Chromatogr. A 1673, 2022: 463054; https://doi.org/10.1016/j.chroma.2022.463054.
5 Frey DD, Barnes A, Strong J. Numerical Studies of Multicomponent Chromatography Using pH Gradients. AIChE J. 41(5) 1995: 1171–1183; https://doi.org/10.1002/aic.690410513.
6 Persat A, Chambers RD, Santiago JG. Basic Principles of Electrolyte Chemistry for Microfluidic Electrokinetics, Part I: Acid-Base Equilibria and pH Buffers. Lab Chip 9(17) 2009: 2437–2453; https://doi.org/10.1039/b906465f.
7 Goldberg RN, Kishore N, Lennen RM. Thermodynamic Quantities for the Ionization Reactions of Buffers. J. Phys. Chem. Ref. Data 31(2) 2002: 231–370; https://doi.org/10.1063/1.1416902.
8 CBER/CDER. Guidance Document: Q8(R2) Pharmaceutical Development. US Food and Drug Administration: Rockville, MD, November 2009; https://www.fda.gov/media/71535/download.
9 Dong DD, et al. Abbott Laboratories. 2012/0238730. Integrated Approach to Isolating and Purifying Antibodies. US Patent and Trademark Office: 20 September 2012; https://ppubs.uspto.gov/dirsearch-public/print/downloadPdf/20120238730.
Based in Worcester, MA, corresponding author Natraj Ram ([email protected]) is vice president of innovation at Thermo Fisher Scientific. He has worked in process development at Takeda, Alkermes, AbbVie, Pfizer, and Cambrex BioScience. He is also the project lead for BioPhorum’s buffer management team and a scientific advisor for BPI events. Adharsh Ravi is a senior in the S program for computer and information systems at Framingham State University in Framingham, MA. The freely available Expert Buffer Designer tool at https://buffer-designer.web.app is not affiliated with Thermo Fisher Scientific.