Current biomanufacturing is driven to pursue continuous processing for cost reduction and increased productivity, especially for monoclonal antibody (MAb) production and manufacturing. Although many technologies are now available and have been implemented in biodevelopment, implementation for large-scale production is still in its infancy.
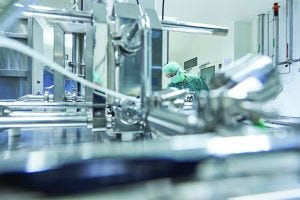
RENTSCHLER BIOPHARMA (WWW.RENTSCHLER-BIOPHARMA.COM)
In a lively roundtable discussion at the BPI West conference in Santa Clara, CA (11 March 2019), participants touched on a number of important issues still to be resolved and technologies that are still in need of implementation at large scale. Below, moderator Peter Satzer (senior scientist with the Austrian Center of Industrial Biotechnology, Vienna) summarizes key points raised in that session. Based on his highlights, BPI asked a number of industry representatives to comment on those points further, and their responses follow.
Requirements for Continuous Downstream Applications
by Peter Satzer
Minimizing Buffer and Hold Tanks: One critical parameter is integration of unit operations on manufacturing shop floors using minimum numbers of buffer tanks and hold tanks. The benefit of continuous manufacturing can be realized fully only if auxiliary equipment for buffer preparation and hold tanks are minimized between downstream operations. Although continuous buffer preparation and reduction of buffer hold tanks are being investigated (and also were the subjects of BPI West presentations), direct integration without the need for hold tanks between unit operations requires further exploration.
Chromatography Operations: Some unit operations such as flow-through chromatography and single-pass, tangential-flow filtration (SPTFF) can be integrated easily into a continuous downstream process scheme at any point because they offer constant inflow and outflow of material. Such operations can be called truly or fully continuous.
Other unit operations — especially bind–elute chromatography — are much harder to implement because they don’t provide truly continuous operation, but rather produce individual elution peaks with fluctuating product and impurity concentrations. Usually the unit operation following such a step cannot handle such changes in flow and concentration directly, requiring inclusion of surge vessels and hold tanks that interrupt the process. This makes continuous periodic countercurrent chromatography (PCC) a unit operation that is harder to implement than other operations such as flow-through chromatography and new, alternative purification methods, including continuous precipitation.
Viral Inactivation: Current implementation of continuous processing in the MAb world could make use of viral inactivation steps as surge vessels. Biomanufacturers could fill parallel vessels periodically and empty them to provide constant flow and product concentration for subsequent unit operations. This might not be possible for all antibody and nonantibody products, however. Alternative purification techniques such as continuous precipitation offer a constant inflow and outflow of material and prevent integration issues.
Residence-Time: Another important point to consider when thinking about continuous integrated unit operations is the residence-time distribution through all unit operations. Understanding of this concept is limited, but it is critical to ensure batch definition and ensuring process robustness. Although a narrow residence-time distribution is preferred for batch definition and to minimize product loss in case of contamination, a broad residence-time distribution smooths process irregularities and leads to a more robust process. The trade-off between those two approaches will need to be discussed in the future.
PCC Chromatography: PCC can be detrimental to our understanding of residence-time distribution because it can split it during an operation. A PCC operation will produce one or two residence-time distribution peaks depending on the state of the operation, and no easy model can be applied. If bind–elute polishing also is implemented using PCC, the resulting residence-time distribution can be split into up to four individual distributions traveling through the system, which complicates decisions about when to discard material. Currently, this issue is too poorly understood and modeled. It either is not addressed, is addressed with wide safety margins for discarding material, or is addressed by implementing a hybrid process that uses batch manufacturing after a PCC unit operation.
Alternative technologies to bind–elute chromatography such as flowthrough chromatography, precipitation, crystallization, and flocculation can offer solutions to those issues in the future to preventing integration problems. Developers of future technologies should aim for a constant mass flow through all unit operations, enabling integration and a seamless downstream process train.
The Roundtable Discussion
Based on the discussion highlights above, BPI invited further insights from several advisors, end users, and supplier companies. Included below are additional comments from Satzer along with responses from participants listed in the box at the left. BPI invites further comments and welcomes submitted manuscripts detailing how your organization approaches these issues.
Capacity Mismatch
We’ve heard recent discussion about a “capacity mismatch” between upstream and downstream aspects of biopharmaceutical drug-substance manufacturing. A couple decades of production improvements have created challenging process streams for separation and purification. Can continuous downstream processes offer a partial solution to this problem?
Satzer: Continuous downstream processes can offer solutions in two separate ways. The first way is to increase downstream equipment use by using equipment 24–7 and therefore increasing downstream productivity. The second way is through a change in mindset, in which up- and downstream operations are integrated directly rather than remaining distinct and separate — which forces them into direct communication. That opens the possibility of solving problems earlier (upstream), where solutions might be easier to achieve.
Holzer: Great improvements have been made in cell-specific productivity, media development, and process design during recent decades, resulting in some cases up to 100× increased product concentration in fermentation broth. The product output of the same upstream installation could be increased proportionally.
However, improvements in chromatography media capacity and membrane performance have not given the same range of productivity increase, instead doubling or tripling it. Therefore, work is needed on process design to overcome the downstream bottleneck. Related design studies easily show the great potential of continuous processing.
Monge: Recent advances in upstream processing have led to processes in which expression titers have increased beyond 10 g/L or even 20 g/L. Availability of concentrated fed-batch options on a pilot and commercial scale has resulted in DSP handling product amounts ranging from 5 kg to 40 kg per batch. On one hand, process intensification efforts have reduced overall footprint and time in the upstream train by eliminating intermediary seeding steps. But this has led to an increased footprint in downstream processing (DSP) that has required some biopharmaceutical companies to switch from single-use to hybrid/stainless steel technologies.
One way to address the challenge is to implement multicolumn chromatography at the capture step to ease the burden or to have continuous DSP with perfusion-based upstream processing (USP) — enabling biopharmaceutical companies to debottleneck this “capacity mismatch” because the amount of product to be processed is distributed daily. The approach also provides high flexibility in product output, reduces the overall DSP footprint, and enables closed processing and cleanroom declassification. With the same scale for clinical and commercial production, the technology transfer process is seamless.
Daumke: Different options can help solve the mismatch. One option would be a single-use centrifuge, and another would be filtration. In the latter case, alluvial filtration can be developed and built as a continuous skid working similarly to a chromatography system. When capacity is reached in the first filter, the system switches automatically to the next filter, and so on.
Faude: Continuous processing contributes to facing DSP challenges in two ways. First, it usually goes in hand with increased automation compared with conventional batch processing. For example, using more sophisticated chromatographic devices allows manufacturing to run efficiently over 24 hours. Second, the continuous processing mode enables higher resin use, resulting in lower buffer amounts needed for processing. Both can have significant impact on costs. Moreover, continuous processing can trigger optimization of simple process steps such as flow-through applications and should push forward the development of resins, membranes, and other technologies that can deliver fast mass transfer.
Buffer Preparation
Whereas continuous upstream processing uses more buffers and media, continuous downstream processing should reduce the number of buffers required for separation and purification operations. Does the end result balance out? And does it make more sense to buy and store premade, ready-to-use buffers for continuous processing — or to make them on demand based on powders and/or concentrates?
Satzer: The final goal has to be preparation of buffers and media based on powders or concentrates. For concentrated buffers (and reduction of different buffers used in the complete downstream stream), efforts have been made already and the first prototypes created (some presented at BPI West). Media tanks especially occupy a significant portion of a shop floor and either take up significant space or have to be refilled regularly (requiring personnel, analytics, maybe cleaning, and so on). Buffer preparation is an essential part of DSP, so development of continuous buffer preparation from powders is fundamental to a fully continuous process with minimal shop-space requirements.
Holzer: Perfusion or chemostat cell culture processing typically need more media while significantly improving plant productivity. Depending on the process design of unit operations in DSP, the amount of necessary buffers and solutions may be reducible.
For example, continuous downstream processing (cDSP) could be achieved by connecting staggered batch operations, but that would not reduce buffer consumption. Yet in the case of countercurrent multicolumn chromatography processes or single-pass TFF, typically more product can be processed with less buffer. It is important to analyze an individual product, plant, and strategy to come up with an adapted, balanced, and cost-effective processing platform.
Cost for buffer preparation, analysis, and storage are significant for recovery and purification steps and can become an operational bottleneck for some plants. That might be not seen during clinical phases, but it becomes evident during commercial production. Cost studies and risk assessments should support decision making. Results often bring about interest in working with concentrated solutions using in-line dilution systems. Currently, technologies that allow buffer or media preparations starting from powders are under development.
Monge: Performing chromatography in multicycle, sequential batches with smaller columns/adsorbers that require a high degree of saturation has led to a considerable decrease in downstream buffer demand. Choosing the right buffer-management strategy would require a company not only to evaluate the buffer demand on a unit operation and process level, but also to evaluate the impact of each proposed preparation and distribution concepts on mobility, adjacency, and room classification.
As far as continuous processing is concerned, choosing ready-made buffers connected at the point-of-use, along with in-line dilution and stream conditioning for steps with low buffer-demand could be an option. So could choosing a buffer-on-demand system with powder/concentrate that eventually will be formulated into buffers and released in real time for steps with moderate to high buffer demand.
Adapting that approach would mean that buffer needs/demands in DSP could be addressed continuously by a modular, intelligent buffer skid that is adjacent (e.g., in close vicinity) to the DSP skid but in a different room. That would eliminate the need for high-volume single-use mixers for buffer preparation and significantly reduce the area required for buffer preparation and distribution.
Faude: Buffer reduction in continuous downstream processing seems unable to adjust to increased media amounts used in USP. Using buffer concentrates is a very interesting possibility for reducing downstream buffer volume substantially, especially when DSP occurs in facilities that are not designed for continuous processing.
Filtration
Continuous filtration can involve problems related to low flux. Does this — or do other challenges — limit its potential in downstream processing? What kinds of technological solutions are needed?
Faude: Prepared backup filters placed in parallel might solve the problem. Switching to a parallel filter could be automatic depending on the flux course. Doing so might make filtration a simpler and better-controllable process step compared with continuous column chromatography.
Satzer: Filtration can be made continuous in two different ways: by exchanging filters or by using membranes in TFF mode (either single pass or not) with regular cleaning and regeneration or. In the end, all filters tend to foul over time in a continuous process and will cease to function at some point. The exchange of filters and continuous filtration can be used (and must be used in the case of dead-end filtration) and has been demonstrated by our group and others for viral filtration. In my opinion, the technology exists and can be used, but large-scale implementation is still missing.
Holzer: Many different filtration principles (e.g., micro-, ultra-, and nanodepth filtration and membrane adsorption) are applied during downstream processing. Most filters are used in a frontal-filtration mode batch process, in which concentrations change over time and reach the maximal limit of filtration capacity. Bioburden reduction, depth, or sterile filters are examples.
Redesign of those filters and unit operations into a tangential continuous process mode often is not of any process and economical interest. Therefore, the most efficient way is to work with staggered-batch or parallel-batch configurations. The flux is proportional to the membrane surface area or the number of filters used.
TFF processes allowing for continuously run unit operations are applied typically to cell separation, buffer exchange, and product concentration. Because the flow of a continuously run operation usually is lower than in a batch operation, technology is available or can be designed properly (for example, in the case of single-pass TFF).
Reducing the number of unit operations during downstream processing — such as by buffer exchanges or concentration between steps — is a great help for reducing cost and improving productivity. This is facilitated by the availability of chromatography media that can accommodate higher salt concentrations and flow rates with more targeted buffer choices.
The integration of viral filtration steps into continuously run DSP lines continues to be challenging. Process models and software could help us further characterize this unit operation, capitalize on historical data, and assist in process design and control. Use of process analytical technologies (PAT) to reveal rapid information about filter integrity or the amount of filtered product are of utmost importance as well.
Monge: Continuous processing created an additional driver to understand further the technical and operational aspects of establishing filtration steps toward manufacturing implementation. The worst-case criteria for batch-mode filtration do not translate to newer process scenarios. Besides a lower volumetric flow and prolonged exposure, significant challenges that need addressing include filter installation, integrity testing, and the impact of process variations and physicochemical feedstock variations.
The challenges mostly involve virus filtration because the number of other filtration steps is decreased in an integrated processing approach. Small, smart surge tanks are one way to reduce many concerns. They can serve to bridge downtime and compensate for process perturbations from other unit operations.
Daumke: In my opinion, the whole liquid flow needs to be optimized to reach the flow needed. Clarification the use of (for example) depth or alluvial filtration requires a certain flow to become effective. Therefore, other options need to be investigated (e.g., continuous centrifuges or TFF).
Chromatography
Continuous bind–elute chromatography involves concerns over residence time and loading capacities. Do you see this as a chance for more flow-through operations to succeed? What about alternative purification technologies such as precipitation/flocculation?
Satzer: Bind–elute chromatography cannot be run in fully continuous mode. Flow-through operations would be preferred, but in many cases they cannot offer the same purification efficiency as bind–elute chromatography. We demonstrated that precipitation/flocculation can yield similar purities to affinity chromatography in bind–elute mode while offering a constant mass flow. So I believe that those technologies (along with flow-through chromatography for polishing) will solve the issues of bind–elute chromatography.
That said, I think that bind–elute chromatography is here to stay. There might be separation and purification tasks for which no suitable precipitation technology exists, and the biopharmaceutical world is conservative when integrating new methods. It will tend to implement more familiar methods (such as PCC), at least in the near future.
Holzer: Control of residence time and loading capacity is important for batch and continuous (countercurrent multicolumn chromatography) bind–elute as well as for flow-through chromatography (where impurities are bound and can break through if residence times and process conditions are not controlled). Such applications require tighter control and more process understanding for continuous bind–elute chromatography because robustness is reduced.
Flocculation and precipitation steps for impurities and target products are applied in several industrial production processes (e.g., impurity precipitation/ flocculation mainly for products expressed in microorganisms and product precipitation in Cohn plasma fractionation). Based on the performance of protein A capture steps for antibodies, it might be difficult in this case to compete with bind–elute chromatography. Such alternative technologies need to be evaluated case by case, taking into account precipitation behavior, solubilization conditions, processing times, product quality, yields, and so on.
Monge: Bind–elute chromatographic operations are by nature discontinuous processes and can become a bottleneck. Other techniques such as multicolumn and simulated moving-bed chromatography can bring resin-based systems closer to a continuous mode. However, the approach requires sophisticated hardware systems with tedious automation and validation needs.
On the other hand, membrane-based flowthrough polishing provides a cost-effective and highly productive alternative to resin-based bind–elute chromatography. Many experts anticipate that eventually all polishing flowthrough steps could be membraneadsorber–based. Current concerns about larger volumes could be addressed with new process schemes. Given that chromatography steps comprise most of the process bottlenecks and complexity in DSP, alternative approaches such as precipitation or flocculation that increase product purity without compromising product quality/activity before chromatographic steps will help streamline the process.
Faude: Independent and continuous processing flowthrough operations are used wherever possible to shorten process times and achieve more economic separations. Even for chromatographies that traditionally were applied in bind–elute mode for MAb purification (e.g., cation exchange), suppliers are beginning to provide new resins designed especially for flowthrough applications.
Alternative technologies are under consideration, but platform processes are still in development. Technologies that can be used more generically will attract greater interest. An example is the recent trend toward considering flocculation technologies to optimize harvest-filter capacity and precipitation for optimizing impurity reduction.
Virus Safety
Low-pH and detergent treatments are difficult to automate, which is necessary for continuous processing. Viral safety is not a single unit operation, but rather is expressed as the cumulative effects of many operations. Does continuous processing bring new challenges to achieving such results? And which viral safety operations will be most difficult to adapt to a continuous approach?
Faude: Low-pH treatment especially needs equipment and technology for appropriate process control. Detergent treatment can be implemented easily from a technical point of view, but detergent removal, potential side reactions with the detergents, and the potential presence of trace impurities increase development and analytical costs. Additional challenges in virus clearance studies for continuous processes are to acquire representative starting materials and addressing ramp-up and shut-down phases as well as transient operation ranges of steady-state unit operations.
Satzer: The first parameter to assess when moving to continuous operation is a new definition for exposure time. Typically in batch processes this is defined as a certain time period, but for continuous operations there is a residence-time distribution (meaning that some molecules stay longer, some not as long in the low-pH environment). It has to be ensured that even molecules with shorter residence times will have adequate viral inactivation. This issue can be prevented by implementing larger safety margins or by using hybrid processes in which viral inactivation remains a batch operation.
Another approach is to redefine the viral inactivation step itself. Historically, this is set (for antibodies) to be around one hour. New research shows that a few minutes are sufficient for total viral inactivation. So in comparison with the current approach, there already is a very large safety margin. I think the greater challenges in viral clearance has to do with filtration, because filters have to be exchanged, and exchanges have to be validated. In general, technologies for implementation of continuous viral safety have been shown at laboratory scale, so I think implementation is rather straightforward for this step.
Holzer: New technologies such the BioSC pilot system (Novasep) allow for perfect control of unit operations such as low pH- or detergent-based viral inactivation, definition of different unit operations (such as staggered-batch, parallel-batch, or continuous multicolumn chromatography), integration of several different unit operations to achieve cDSP, and the possibility for different process scheduling of simultaneously run unit operations. Control and integration of viral filtration into cDSP needs more development.
Monge: Virus inactivation (VI) is considered one of the two orthogonal steps required for virus safety in a biotherapeutic production process. Making the process automated and continuous poses several challenges. Various industry groups have adopted different approaches — including a tubular plug-flow reactor, a continuous stirred-tank reactor, and column-based reactor — and successfully demonstrated the implementation.
The challenge currently is a lack of standardized and validated viral inactivation strategy at scale or in a proven scale-down model. With continuous processing, the significant problem is dividing an integrated operation into discrete unit operations for virus clearance testing. Virus filtration is a robust orthogonal method in downstream applications that is amenable for use in a continuous process. Issues that need addressing here are process variation/ perturbations and physicochemical feedstock changes. However, in both cases, validation-scale challenges are real and need to be understood before implementing a full-scale continuous process.
Residence Time
Can you describe the distribution of residence time across a downstream process and how it affects products and the bottom line? Is this a problem for continuous operations, or is there a way to address it?
Satzer: Residence-time distribution through complete processes is poorly understood. Research has been limited because batch processing does not have or need a description of residence-time distributions. This question is unique to continuous processing and therefore relatively new in the biopharmaceutical world. Models are available for some unit operations and for some molecules, but to fully understand all residence-time distributions of all unit operations and impurities and products, we still need a lot of research. To assess the question for product quality, we have to define what components to track, because not all have the same residence-time distribution. For instance, aggregates tend to elute at the front and back of a protein-A elution peak, creating different residence-time distributions for the product and for the impurities. Both have to be known before you can make any decision on product quality at the end of your process.
Only the generation of true “digital twins” with process models for all involved molecular species (such as product, impurities) can solve this completely. But discussion has only just started on what parameters have to be tracked and what might be omitted from those models.
Monge: Processing time ranges need to be characterized for unit operations and integrated downstream processes to ensure product stability and control of impurities. Based on the criticality of product residence time at a specific unit operation, additional studies might be necessary to define control strategies (e.g., tight control of pH and time for low-pH virus inactivation). The characterization of residence-time distribution becomes even more important in cDSP because it serves also as information for material traceability and helps during impact analyses in case of investigations.
Timing/Transition
It’s a general belief that the transition from batch to continuous processing should be made as early as possible in product development. If you consider the challenges that come with transitioning too early and too late, where does the “sweet spot” typically come between them?
Satzer: At the moment we face some issues in process development. In my opinion, the switch starts with the cell line. Current cell lines are adapted for fed-batch high production, so they might not be at peak performance for use in perfusion cultures. We experience quite drastic changes in impurity patterns and therefore downstream development when switching from batch-produced material to perfusion material.
I think the earlier that transition is made, the better, and one limiting factor (availability of small-scale equipment for running perfusion) has been lifted recently with combining the ambr system (from Sartorius Stedim Biotech) with automated screening of chromatography performance using Robocolumns technology. Additionally, this might depend a great deal on the product produced.
For antibodies, we know that protein A will perform, regardless of what material you use, and minimal adjustments are necessary when the feedstock changes. But for products that do not have a high-performance affinity capture option, providing material that adequately resembles that produced in pilot/large-scale DSP as early as possible is crucial for process development.
Holzer: Typically, process design of countercurrent chromatography or membrane steps, plug–flow reactions, in-line dilution (ILD), and so on need additional developments and dimensioning studies. Therefore, this development stage would be perfect for implementation. The results of these studies allow also for specifying processing equipment requirements. However, changes that mainly concern process scheduling and not development of the unit operation to achieve cDSP could be implemented later in product/ process development. In some cases, facility-fit challenges might be addressed with implementation of in-line buffer dilution or continuous multicolumn chromatography for one single unit operation even for commercialized products.
Faude: The development of continuous processing seems to be more time consuming unless you have a robust platform for some types of molecules. But that could conflict with the importance of time to [reach] toxicology testing and time-to-market considerations in early phases. The transition to continuous processing might be during late-stage development with a stronger focus on robustness and economical aspects.
Monge: Speed to clinic and market are significant drivers for biopharmaceutical companies. In this context, it would be better first to develop a batch-based platform process and manufacture product for clinical, process validation, preapproval/registration, and commercial scale. That would enable companies to enter the market and cater to its needs quickly without having to worry about uncertainties that continuous processing could bring from quality, manufacturing, automation, and regulatory perspectives. A parallel work stream needs to be in place where the same product is developed using a continuous process along with evaluation of the transition from batch to continuous manufacturing and identifying a bridging strategy. Once that is completed, then a postapproval change to a continuous process can be made by filing a prior approval supplement (PAS) as outlined by the US Food and Drug Administration (FDA) in its latest draft guidance covering quality considerations for continuous manufacturing.
S. Anne Montgomery is cofounder and editor in chief of BioProcess International. Peter Satzer, PhD, is a senior scientist with the Austrian Center of Industrial Biotechnology (ACIB), Muthgasse 18, A-1190 Vienna AUT; +43 1 47654 79114; petersatzer@ acib.at; and lecturer at the University of Natural Resources and Life Sciences, Vienna, Muthgasse 18, 1190 Vienna AUT, email [email protected].