Toward Nonantibody Platforms
November 1, 2012
Monoclonal antibodies (MAbs) remain the largest segment of the biopharmaceutical market, but they are not the only recombinant proteins in development. Remember that the first biopharmaceutical approved for sale was recombinant insulin — a hormone — back in the 1980s. And proteins aren’t the only recombinant biologics. The sector has expanded since then to include gene therapies and viral vectors, vaccines, and even cells and tissues. Companies around the world are developing such products for cancer, neurological, infectious disease, metabolic, autoimmune, and cardiovascular disorders, to name just the most prominent. And although MAbs are finally fulfilling their “magic bullet” promise, many other approaches are becoming available to drug developers targeting those markets — and others.
Meanwhile, funding challenges are increasing emphasis on manufacturing and development efficiencies. Even though total funding of the biotechnology industry has rebounded since the 2008 recession — from about US$13 billion for the United States industry in 2008 to about $21 billion in 2010, for example — a growing share of that money is going to the less risky investments. According to Ernst & Young’s 2011 Beyond Borders report, that means mature and already-profitable companies are taking a larger portion of the financial pie.
At the same time, the average number of drug approvals per year has decreased: from about three dozen in the United States from 1996 to 2004 to under two dozen for the years since. And even though markets are opening up in China, India, and other countries, the cost of doing business on a global scale makes it no easy task to reach them. So biopharmaceutical companies need to curb the rise of development and manufacturing costs. Single-use technologies are helping with the latter in large part. And platform technologies have helped antibody makers shorten development times by starting out with certain rules of thumb — rather than trying out hundreds of available purification technologies, for example, in many different combinations to find what works best for every new product candidate.
Do nonantibody makers have similar options when it comes to their own process development work? As is so often the case in bioprocessing, the answer to that question is “It depends. ..” on the product class; on the expression system; and on the regulatory history of the company, process, and type of molecule.
The Antibody Platform
For over a decade, downstream processing for MAbs has followed a familiar pathway: harvest and clarification (typically involving depth filtration, centrifugation, and/or microfiltration); antibody capture (using high-yield protein A chromatography); a low-pH viral inactivation treatment (also known to precipitate out host-cell proteins, but with potential product stability issues that must be addressed in process development); ion-exchange polishing chromatography (for removing aggregates, endotoxins, unwanted proteins, and even viruses) followed by solution concentration and buffer exchange (usually involving tangential-flow membranes); and ultrafiltration, diafiltration, and/or nanofiltration for reducing any remaining viruses and bioburden.
Antibodies are typically produced using animal cell culture, most often involving Chinese hamster ovary (CHO) cells. Together, CHO expression and protein-A– based purification represent a powerful manufacturing platform that has worked well for a number of MAb products on the market. Regulatory reviewers in the United States, the European Union, and beyond are comfortable with this platform — which encourages companies to use it. The platform provides a basic rule-of-thumb for process developers to begin with, something they can then adapt to their own particular products. For those who might be nervous about inviting heightened regulatory scrutiny of their processes and products, it provides some reassurance.
Genentech’s vice president of bioprocess development, Brian Kelly, made the case for the MAb platform approach in a review article that he wrote while he was director of purification process development at Wyeth BioPharma (1). He pointed out that companies seeking to solve current and future process bottlenecks might be looking at other technologies, considering the possibility that conventional unit operations could limit future advancements. He evaluated potential limitations of process chromatography and filtration using commercially available resins and membranes (for a process making 10 tons of MAbs per year at a single plant) in two chromatographic and two ultrafiltration steps. The conceptual process was modeled after a platform purification train that generated 10-kg batches in clinical production. “Based on analyses of cost of goods and the production capacity of this very large-scale purification process,” he concluded, “it is unlikely that nonconventional downstream unit operations would be needed to replace conventional chromatographic and filtration separation steps, at least for recombinant antibodies.”
MAbs are actually less expensive to make than are most other recombinant proteins — partly thanks to platform technologies — but they are also required in much higher doses (2). All that said, the MAb platform is not a “done deal.” Production titers have advanced to the point at which commercial MAb quantities can be produced in 1,000-L bioreactors or smaller — but such high-concentration supernatants are posing a challenge to traditional approaches. Many experts are now saying that the platform approach has stifled innovation.
Pete Gagnon of Validated Biosystems says that biosimilars will drive technology change, particularly away from protein A (3). Process improvements could allow follow-on manufacturers to become more competitive, perhaps even implementing changes that demonstrably improve their product safety over the originals. That could lead regulatory agencies to tighten specifications, which might pose as much of a potential threat to innovators as revenue lost to competition. If even one biosimilar manufacturer makes a significant improvement with a nonplatform approach, then the original manufacturer loses its “home field advantage.” Gagnon observes, “Platform purification has been discouraging innovation for more than a decade, and that kind of dependence on an aging technology can be dangerous. Given the stakes, this is bound to be an emotional issue, but it can’t be ignored. The longer the platform stays in place, the more vulnerable it becomes, and the more disruptive the eventual shock if a more competitive technology appears.”
Intensive efforts continue industry wide to develop major improvements, as discussed in a recent review addressing technology trends in the field (3). Most of those improvements enhance the current platform approach without fundamentally changing it. These include high performance tangential flow filtration (HPTFF), improvements in stationary architecture (diffusive and perfusive microparticles, adsorptive microfiltration membranes, monoliths, and nanoparticles), and continuous multicolumn systems. The major exception is aqueous two-phase extraction (ATPE), which seeks to replace protein A as the primary capture method. ATPE claims to already support better economics and lower environmental impact, but it hasn’t shown enough benefit to justify the physical cost of implementation. Many developers have focused on head-to-head protein A replacements, for example with alternative bioaffinity or mixed-mode chromatography ligands, but these carry the same throughput limitations as protein A. Other capture options await significant enabling developments.
“Still,” Gagnon notes, “you can’t tell what may be right around the next corner. Protein A
was known for decades before it transformed the field. With all the recent advances in alternative technologies, it could easily happen again.”
Whatever new technology eventually arises to challenge the current platform, it remains to be seen which companies will be courageous enough to take the leap of faith. Will that leap pay off — for innovators, biosimilar manufacturers, or both — or will someone have to fail before the first success transforms MAb manufacturing? Will a new platform appear on the scene? Only time will tell.
Innovations in the biomanufacturing industry are traditionally incremental in nature (refinement of existing technology), but occasional disruptive inventions (exploiting novel technology or knowledge) can drive rapid and adaptive change. (4)
What’s Not an Antibody?
Antibodies are the fastest growing segment of biopharmaceuticals, by themselves representing about a third of recombinant protein sales; all other proteins combined make up the other two-thirds (5). Nonantibody product classes in development also include vaccines, gene therapies and viral vectors, and cell/tissue therapies — but mostly, other proteins/peptides (e.g., hormones, enzymes, cytokines, growth factors, recombinant plasma proteins, and subunit vaccines). The pipeline includes antibody fragments and fusion proteins as well. Some of those nonantibody products are expressed by mammalian cells; others are produced through microbial fermentation.
When expressed in the form of bacterial inclusion bodies (IBs), aggregated proteins must be solubilized and refolded as part of downstream processing. But separating and protecting those recombinant proteins from potential contamination within the bacterial cells could be considered a purification step in itself. Given appropriate technologies for handling them, IBs thus could be considered the basis for a platform downstream process — even on par with protein A, should such technologies prove powerful and economical enough.
Most biomanufacturing costs are fixed — e.g., utilities, labor, overhead, and capital — and project risk and capital cost both decrease as product development progresses, so emerging technologies that reduce capital investment and/or speed a project to those risk-reduction clinical milestones are inherently attractive (2). This applies as much to nonantibody products as to antibodies.
Whereas protein A has provided a useful affinity capture platform for MAb purification, says Steve Barton (chief executive officer of ProMetic BioSciences Ltd. in the United Kingdom), “bioequivalent platforms for nonantibody molecules have proved elusive” (5). He points out that synthetic ligands created using computational chemistry and ligand library screening can be targeted for “almost any protein. Such ligands provide a means of capturing and purifying nonantibody proteins and are also very robust and can be reused for many purification cycles.” Suppliers generate synthetic affinity ligands by screening general ligand libraries, modeling known binding compounds, or identifying potential binding sites on a protein of interest to model complementary ligands in silico. Barton says that techniques such as molecular docking provide useful tools for predicting how potential ligands could interact with a particular region on a given protein, and virtual screening algorithms can be used to preselect potential candidates for synthesis and in-vitro screening. Rationally designed chromatography media — or possibly membrane adsorbers — could form the basis of purification platforms.
Antibody fragments are one of the next important classes of protein-based biotherapeutics. According to one recent report, the growing diversification of fragment types could lead to regulatory approval of some products with efficacy and safety profiles that are better than those of full-sized MAbs in the coming decade (6).
Because fragments lack the protein-A–binding Fc-region, a platform approach to purification would be challenging. Furthermore, antibody fragments are a diverse group of molecules in themselves — e.g., Fabs, scFv, and domain antibodies — based on either κ or λ subclasses.
“Having a small designed toolbox of powerful affinity capture chromatography media still offers the possibility to develop a purification platform,” says Mats Gruvegard (antibody program manager for GE Healthcare Life Sciences in Uppsala, Sweden). “For antibody fragments, process development scientists already have the possibility to reduce process development time by using a platform approach based on affinity ligands, either with well-established technologies (such as recombinant protein L) or novel ligands based on camelid antibody fragments.”
Other Proteins: Most biopharmaceuticals approved for market in the 20th century were nonantibody products: e.g., human insulin in 1982, human growth hormone in 1985, and erythropoietin in 1989 (all hormones); tissue plasminogen activator (a serine protease) in 1987; granulocyte and granulocyte-macrophage colony stimulating factors in 1991 and interleukin-2 in 1998 (cytokines); and glucocerebrosidase in 1994 (among other therapeutic enzymes from Genzyme Corporation, which has made them a specialty). They were all developed in the early years of trial-and-error process development, when laboratory purification processes were adapted for manufacturing plants. Their processes were validated according to the old familiar paradigm based heavily on equipment and test qualification, lot-release testing, and so on. Manufacturing costs were considered to be far less important than safety, efficacy, and quality of products. But later biopharmaceuticals would benefit from the lessons those pioneers learned.
The advent of quality systems, process analytical technology (PAT), and quality by design (QbD) in bioprocessing has brought on a focus on risk management. Economic changes have increased emphasis on cost management, as well — adding a fourth leg to the safety–efficacy–quality stool. Both cost and risk are partly addressed through platform purification technologies. In fact, the MAb platform exists thanks to the binding chemistry between protein A and the Fc region of antibodies. So it seems that other classes of proteins might have a similar physicochemical or structural aspect that could lend itself to a similar approach. Unfortunately, that is a more difficult proposition than it would seem.
Cytokines, for example, belong to a large and diverse family of signaling molecules from many different types of cells. Structurally they are classified into four categories, and functionally they are divided into two types. However, they are also signaling molecules — which means that each one has a receptor counterpart that appears on the surface of certain cells. Those receptors have gained attention partly because some are linked to particularly debilitating immunodeficiencies. And maybe those receptor proteins (classification work on them is ongoing) could be used in separation and purification as well as considered for pharmacotherapeutic targets.
The global market for interferon alpha alone is currently estimated to be worth $2.5 billion (7): “The rising incidence of certain cancers and viral hepatitis, in addition to ongoing investigations of novel therapeutic applications are increasing the needs for human recombinant IFN-α2b. There are also significant opportunities for growth in the generics market as IFN-α2b recently reached the end of its patent protection.”
Recombinant human interferon is currently made through fermentation of Escherichia coli. So it requires refolding in downstream processing to restore the molecule’s correct and active structure as well as a PEG
ylation step to make up for the fact that bacteria cannot glycosylate the protein (7). Ultimate recovery rates run around 20% or less, and processing can also lower specific activity and even lead to products that can trigger an immune response. Some researchers are developing mammalian expression systems for production of properly glycosylated cytokines — among them, Yves Durocher’s group at the National Research Council in Canada working with the HEK293 cell line (7):
The volumetric productivity and recovery of HEK293-produced IFN-α2b is comparable to some productions of nonglycosylated IFN-α2b performed in E. coli and in the methylotrophic yeast Pichia pastoris. The volumetric production of IFN-α2b with the selected HEK293 clone largely and reproducibly exceeded 200 mg/L in a simple fed-batch, serum-free culture maintained for only seven to eight days. The simple, rapid, and cost-effective method developed for the recovery of HEK293-produced IFN-α2b yielded 98% pure interferon with a recovery greater than 75%. In addition, IFN-α2b productivity of this clone remained stable in the absence of selection pressure for more than nine months in culture.
Does that represent the birth of a new platform? The technology is currently available for licensing, so the answer remains to be seen.
Cytokines are a diverse and challenging class of proteins (8). But some of them are known to interact with antibodies — making antibody-based affinity purification an option. In fact, H. Fai Poon (R&D director at Hisun Pharma in Princeton, NJ) points out that cyotokines (and other proteins) could be engineered to include the Fc region of an antibody. That allows for protein A to be used as a ligand. “Affinity chromatography (AC) would thus continue to be the preferred platform technology for downstream purification,” Poon says. “I think that most platform technologies will involve tagging proteins (especially for cytokines or hormones).” Antibody fragments — when used as therapeutics — can also benefit from the historical familiarity that MAbs present to regulators and process developers alike.
The 20 standard amino acids can combine in millions of ways — and then add sugars or other molecules to further complicate matters — to produce billions of different proteins in the natural world. Such vast combinations make proteins and peptides difficult to classify (see box, next page). And the proteome of most organisms will be even more complex than the genome — suggesting that proteins and amino acids are really the basis of biodiversity on Earth. However, whether they’re enzymes, hormones, cytokines, or others, proteins bind to receptors for their function. Those receptors thus have obvious potential as affinity ligands. But there are many more purification technologies that operate based on charge (remember that the net charge of a protein molecule can be manipulated by changing the pH of its environment), hydrophobicity, solubility, and size. As is so often and increasingly the case, it comes down to how well you understand a molecule in development — and how well your expression system builds it. Part of process development is researching “prior knowledge” (9), so once a precedent is set by one company, others are likely to consider following that lead at least. And that’s how platforms come about.
“I believe all biotherapeutics are amenable to platform technologies,” says Poon. “However, there is more incentive to develop platforms for antibodies” simply because they represent the largest and perhaps most understood class of therapeutic protein.
Vaccines are probably the next most diverse class of biologics. They may take the form of live, attenuated, or killed whole viruses or bacteria; acellular subunits (microbial proteins); toxoid and conjugate molecules; and even nucleic acids; as well as immunotherapeutic versions of these (10). As recombinant technology has made inroads with the vaccine industry, newer products are trending toward subunit proteins and nucleic acids. The insect-cell–based baculovirus expression vector system (BEVS) is becoming popular for viral vaccine production.
One other promising expression system — especially for producing bacterial proteins — is the Pseudomonas fluorescens bacterial system from Pfēnex Inc. That company uses a purification platform based on ion-exchange chromatography for capture and hydrophobic-interaction chromatography for polishing, followed by buffer exchange and lyophilization (11):
Vaccine antigens and carrier proteins can be produced in Pfēnex expression technology strains at high titer and quality (full-length, very low degradation), which aids purification process development. Employing ion exchange as primary column increases purity and reduces endotoxin, while allowing additional columns to be added without need for buffer exchange. Leveraging available information (such as theoretical pI) to tailor purification methods to each protein reduces time to reach final process. This approach has been used to purify a variety of vaccine antigens, with the intent of developing these processes further to produce GMP-grade vaccine components for vaccine developers globally.
Another company has put forth a fusion-tag purification platform for vaccines (and other proteins) based on the small (100 amino acids) ubiquitin-related modifier (SUMO) protein. LifeSensors, Inc. has shown that fusing SUMO to a protein of interest can enhance expression, improve solubility, and provide for a generic purification protocol based on immobilized metal-affinity chromatography (IMAC). Certain proteases recognize the tertiary structure of SUMO and precisely cleave it away to release the protein of interest (12). And this is just one example of a fusion-tag–based system.
With the potential to reach $17.4 billion by 2018, the vaccine market is dominated by a few large players — Pfizer, GlaxoSmithKline, Novartis, and Sanofi — even as entrepreneurial companies appear on the scene with new and innovative technologies (13). The market is broad-reaching and includes both prophylactic and therapeutic products addressing conditions as far-ranging as human immunodeficiency virus (HIV) and other infections, Alzheimer’s disease, and cancer. As far as protein-based vaccines are concerned, platform possibilities are there. But they depend on the complexity of the molecules and how they’re expressed. Fusion tags and multimodal chromatography are probably among the most promising approaches — as they are with any other recombinant protein products.
Classifying Proteins Is Far from Straightforward
Solubility: Early in the 20th century, proteins were identified as polypeptides made up of many amino acids. The first attempts to classify them were based on chemical and physical properties because their biological functions had not yet been established. Proteins were classified according to their solubility in a number of solvents. Even though such classification is outdated (proteins with different structures and functions can have similar solubilities — and vice versa), some associated terms remain in use. For example, albumins are soluble in water and in water half-saturated with ammonium sulfate; globulins precipitate through half-saturation with ammonium sulfate. Pseudoglobulins are soluble in salt-free water; euglobulins are insoluble in such water. Naturally conjugated proteins are complex molecules with nonprotein moieties: e.g., glycoproteins, lipoproteins, phosphoproteins, chromoproteins, and nucleoproteins. Many such early protein classes overlap.
Biological Function: Modern molecular biologists prefer to classi
fy proteins according to their biological functions. This approach is not ideal either because one protein can have multiple functions. It works well enough for therapeutic proteins, which don’t enter development without some understanding of their function. Enzymes, cytokines, hormones, proteases, and blood factors are all examples of classifications based on biological function.
Structure: The online Structural Classification of Proteins database (http://scop.mrc-lmb.cam.ac.uk/scop) describes proteins in structural and evolutionary terms:
All-alpha proteins are made up of domains consisting only of α-helices.
All-beta proteins are made up of domains consisting only of ß-sheets.
Alpha-and-beta (α/ß) proteins mainly consist of parallel beta sheets (beta-alpha-beta units).
Alpha-and-beta (α+ß) proteins mainly consist of antiparallel beta sheets (segregated alpha and beta regions).
Multidomain proteins are made up of folds with two or more domains that belong to different classes.
Membrane and cell-surface proteins and peptides (not including proteins from the immune system) are small proteins, usually dominated by metal ligand, heme, and/or disulfide bridges.
Newer, less recognized classes include coiled-coil proteins; low-resolution protein structures (peptides and fragments); and designed proteins (experimental structures of proteins with essentially unnatural sequences).
Within those structural classes, proteins are further organized according to how they fold, then superfamilies and families, as well as species. For example, human trypsinogen is a barrel-shaped all-beta protein consisting of two domains with the same fold, part of the trypsin-like serine protease superfamily, which is a type of eukaryotic proteases (family) found in humans (species).
(HTTP://EN.WIKIPEDIA.ORG/WIKI/STRUCTURAL_CLASSIFICATION_OF_PROTEINS_DATABASE)
Gene Therapies: Some vaccines may also be considered gene therapies — if they happen to be DNA or RNA molecules that may or may not be delivered by way of viral vector technology. The gene therapy market has had a rocky road so far. But Global Industry Analysts of San Diego, CA, released a report this year forecasting the global market to reach a $794 million valuation by 2017. “Key factors driving growth include rising demand for new and effective therapies for cancer treatment in addition to completion of human genome project, rising incidence and prevalence of cancers and other critical diseases, and prospective launch of gene therapies in major global markets” (14).
With only a few base-pairs (compared with the 20 amino-acid building blocks of proteins), the characteristics of nucleic acids are strangely predictable — despite the variety that they present for storing genetic material. For example, H. Fai Poon explains that DNA molecules are negatively charged, so anion-exchange chromatography has been widely used to remove DNA contamination from protein therapeutics. “Lectin has also been used as an affinity ligand for binding DNA,” he says. And what you use to remove DNA or RNA from a protein product stream can work just as well to purify those nucleic acids as products themselves.
For some viral vectors, heparin affinity chromatographymay form the basis for a purification platform (15). Heparin, a relatively inexpensive generic affinity ligand, captures many different types of viruses including adenoassociated viruses (AAV), gamma retroviruses, lentiviruses, herpes simplex virus, and vaccinia viruses that may be used as vectors. Ion exchange is useful as well. A biospecific interaction frequently exploited for virus purification is that between a matrix-bound receptor and a viral surface ligand. And membrane adsorbers (e.g., from EMD/Merck Millipore, Pall Life Sciences, and Sartorius Stedim Biotech), hydroxyapatite multimodal chromatography (e.g., from Bio-Rad Laboratories), and monoliths (e.g., from BIA Separations) offer improved flow rates and capacities for viral vector purification as well as many other product classes. Fusion tags such as His6 endow viruses and proteins with a high affinity for immobilized nickel ions. Viral particles can also be purified by exploiting a specific interaction between biotin and avidin.
Charles Lim (business development VP at BIA Separations Austria) says that a platform approach is amenable to plasmid DNA production. His company has developed one for large-scale DNA vaccines and gene therapy production. He says that up to 48 grams of (sc)pDNA can be produced per cycle using 8-L columns in97% purity and >80% yield of product “meeting regulatory requirements and much lower cost than traditional chromatgraphic methods.” The approach includes two monolithic chromatography steps: a CIM DEAE column followed by a CIM C4 HLD column. He calls those monoliths the “enabling technology” because they are designed for large-biomolecule purification at high flow rates, “with dynamic binding capacity for pDNA as high as 9 mg/mL unaffected by flow rate.” The platform also includes an small (0.1-mL) CIMAC analytical pDNA monolith column that can be used for real-time in-process monitoring (in
Cell Therapies: In a recent BPI article, Barry Rosenblatt of SME Biotech Consulting drew a clear apples-to-oranges comparison of the regulatory and processing approaches for recombinant proteins and cell therapies. Downstream processes for protein products broadly involves harvest, capture and polishing chromatography steps, and viral inactivation and filtration. By contrast, isolation and culture of therapeutic cells leads into centrifugation, counting and resuspension of those cells into an administration buffer (with or without a cryopreservative), then vialing and possibly freezing (16). This is to all intents and purposes a platform that works for any cell type.
Platform Possibilities
I’ve discussed the platform purification concept with experts from industry and academia, who have weighed in on the “pro” and “con” sides of the topic. Pennsylvania consultant Dave Wareheim says, “Platforms minimize pain. Given a class of molecules, if I fix the steps from phase 1 through phase 3, the expectation is that minimal pain will be placed on the commercial plant and equipment/room adaptations, and batch losses will be minimized if QbD is implemented before phase 1. Time to market is minimized, which is hugely important if patent protection is 12 rather than 17 years.”
Wareheim says the real “platform” these days is a multiuse, multiproduct facility based as much as possible on single-use technologies. “Now,” he explains, “you can plug-and-play several platforms for smaller upstream areas, scale-out when scale-up is maxed out, smaller and cycled downstream operations (combining chromatography and filtration steps), and minimized holding. This new ‘platform’ should beat the pants off any fixed-asset system and has built-in risk assessment for commercial-scale manufacturing that is both small and flexible.” Wareheim is one of many experts who see depth and other forms of filtration replacing centrifugation overall.
Tim Clayton (CMC technical director at Janssen Alzheimer Immunotherapy in Switzerland) says, “In my experience, the use of platform technologies is widespread and product-type–specific. It doesn’t mean that these are the best technologies, but that they are fit for purpose. Posttranslational modification of proteins can have a big influe
nce on the choice of technologies, so research and early development decisions affect QbD.”
William G. Whitford (senior manager for the bioprocessing market cell culture and bioprocessing at Thermo Fisher Scientific) also believes that other categories of products can be subject to their own platform approaches. “Differentiators include size and structure of the molecule, previously accepted (or otherwise desired) CMC approaches, orchestrating/coordinating the upstream and downstream technologies, and considering the potentially significant divergences in annual product mass and/or scheduling. It seems to me that because dosage and indication dictate the annual amount needed of a given product, they (beyond pure capability) could significantly influence the manufacturing process format and mode chosen.”
The newer the expression technology — e.g., plant-based vaccine production — the less amenable it may be to downstream platform approaches. This is due not only to regulatory unfamiliarity with such systems, but also a general lack of experience within the industry. Every new expression system faces such hurdles — no matter how impressive it may be. Platforms arise out of building knowledge bases and understanding.
Konstantin Matentzoglu of Trenzyme GmbH in Germany emphasizes that point. “If the protein is a known therapeutic such as Factor VIII or epoetin, then market approval is rather straightforward. By contrast, going into clinicals with a new class of biopharmaceutical can make risk assessment difficult. It pays to involve the respective authorities early on.” For example, the European Medicines Agency (EMA) and the US Food and Drug Administration (FDA) provide expertise and advice for companies that approach them in the preclinical phase of development.
Sancha Salgueiro (vice president of business development at contract researcher ExpreS2ion Biotechnologies in Denmark) thinks “manufacturing may be easier [for antibody products], and some impurities and stress tests are probably faster to set up” than for nonantibodies. But she says that the clinical path is daunting for both, “with the possible exception of phase 1, where toxicity indicators have been developed for the common frame of MAbs.” As always, she says, it will come down to a case-by-case decision. “The difficulty will depend on the molecule. In terms of assessing risk, MAbs are perceived as an understood risk, rather than the more general risk for all molecules.”
Nick Hutchinson (market development manager at Parker Hannifin in the United Kingdom) provides a supplier perspective. “Undoubtedly antibodies raised to specific molecules can be linked to resins and used as capture-chromatography steps. If there are common binding sites within a group of molecules, then in principle this would be a generic capture step for a given product family.” But he points out that this can be quite an expensive option because of the need to manufacture small quantities of high-quality ligands for use as consumables within a manufacturing process. “Hormones, with their role as communication molecules within the body, will bind to specific receptor molecules that could be immobilized onto resins and that interaction be used in an AC step.” He warns that the actual resins themselves could not be used among products within a given family, however, because of the risk of cross contamination. “Another solution for proteins is to engineer in a polyhistidine tag the use of a suitable chromatography step to capture tagged proteins. This would give a high yield and purity.”
Some manufacturers have successfully developed protein-A–free platforms for antibodies already, says Hutchinson, so those should be feasible for other proteins as well. “It is not necessarily the case that a low-yield first column step must be accepted. Switching to an AEX or HIC step can ensure high yields but not necessarily purity. It becomes necessary, therefore, to invest heavily in optimization of subsequent purification steps or to add a step for achieving the final product purity specification.”
Hutchinson also points out that the large sizes of DNA molecules and virus particles make resin chromatography inefficient for them. “So use of membrane adsorbers or related technologies makes sense for the platform processes of such molecules.” And he says that even if the conditions under which adsorbers are operated vary, the same equipment could be used throughout a facility. “There is room for improvement in dedicated single-use skids for membrane adsorbers, which would help enable platforms of this type. It is an avenue that we’re actively pursuing.”
For BPI’s 2008 BioProcess International Conference and Exhibition guide supplement, I asked Tim Herrmann (process development scientist in global biological development/purification at Bayer HealthCare LLC) what would be the characteristics of an ideal purification platform (17). “In the sense of a cascade of predefined purification steps ready to be plugged into the purification train,” he said, “an ideal purification platform should be robust in terms of its applicability to the whole pipeline of upcoming drug candidates. Without requiring more resources than about one full-time employee and a timeline of several days to a few weeks, it should be possible to adjust the platform for a current drug candidate while delivering acceptable to good yields with no cutbacks in product quality and purity.”
For different types of proteins, Herrmann said, “a purification platform would be a predefined catalog of process development steps on the experimental side as well as on the data analysis and prediction/simulation side.” Although requirements for resources in a cascade of “plug-in” purification steps would be high, “the overall amount of resources — and especially development time — can still be significantly reduced.”
Platform approaches have become a key element of industrial and antibody downstream process development activities. Despite the wide range of separation technologies available, chromatography remains the most powerful option (18). For the versatile portfolio of nonantibody drugs, a generic and predefined column cascade has yet to be described and accepted. Some experts wonder, however, whether a platform approach really is something to be desired — not only outside the MAb world, but for antibodies themselves.
One Vendor Offers Free Advice
GE Healthcare published a free handbook in 2007 on Purifying Challenging Proteins, which you can download at www.gelifesciences.com/gehcls_images/GELS/Related%20Content/Files/1336168762999/litdoc28909531_20120505001646.pdf.) Chapters focus on membrane proteins, multiprotein complexes (protein–protein interactions), and inclusion bodies; appendices provide focused information on chromatographic methods and techniques, automation, and tagged proteins, as well as data conversion tables.
“The need to handle and study these more difficult groups of proteins is clear, given that
∼30% of a typical cell’s proteins are membrane proteins, and more than 50% of the current drugs on the market exert their actions via membrane proteins
while carrying out their enzymatic, structural, transporting, or regulatory functions, proteins most often interact with each other, forming multiprotein complexes
a large proportion of normally soluble proteins that are overexpressed in E. coli end up as incorrectly folded and insoluble protein in inclusion bodies.”
Also in 2007, the company published a guidebook on Purification Technol
ogies for Vaccines and Vectors using the company’s products and technologies. Download it at www.gelifesciences.com/gehcls_images/GELS/Related%20Content/Files/1314774443672/litdoc28908405AB_20110831091026.pdf. Chapters focus on viral vaccines (subunit and whole), gene therapies, and viral vectors.
Some Caveats: The Importance of Innovation
Marco Casteleijn (a postdoctoral researcher at University of Helsinki in Finland) points out that it’s hard to be universal, especially where biomolecules are concerned. “Chemical conjugation in combination with cell-free protein synthesis is what we are working on,” he told me, “but these technologies are just now finding laboratory application.”
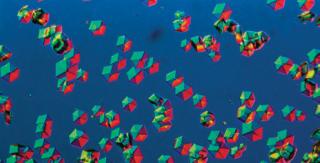
Connect and Learn in 2013 Insulin crystals ()
Consultant Barry Rosenblatt says that there are degrees of “platforming” in all bioprocesses. “As soon as a PD group gains experience with a particular mode of operation,” he points out, “it becomes part of their ‘tool kit’ for all subsequent processes.” But he also says that no “cookie-cutter” or universal process exists (or could exist) for any class, including MAbs. “However, the concept of stringing together known and vetted processing steps to minimize development time applies to all biotherapeutics because this is key to reduction of the cost for a new entity. The caveat, of course, is to prevent squelching creativity and innovation” because they too can reduce costs and provide for better products.
Mukesh Mayani (a research scientist in drug development and downstream purification at Therapure Biopharma Inc.) also pointed out that some analogy in process steps can be found, especially from the middle to final purification unit operations among nonantibody products. However, pretreatment and early processing can vary depending on intra- or extracelluar production, the type and range of impurities, and so on. “I would agree that platform processes are a good starting point for development but may not be economic for routine production processing. Innovation can beat established alternatives!”
“Antibody purification seems to be safely ensconced in a platform,” wrote Duncan Low and colleagues in a 2007 review article (19). “However, natural evolution compels us to peer into the future. This is driven not only by a large projected increase in the number of antibody therapies, but also by dramatic improvements in upstream productivity, and process economics. Although disruptive technologies have yet escaped downstream processes, evolution of the so-called platform is already evident in antibody processes in late-stage development.”
If antibody developers — with their “safe” and established platform — are thinking outside the box these days, perhaps that will serve as a cautionary tale for makers of nonantibody products. Platform technologies are worthy of consideration and development — and many could prove useful and versatile for innovative biologics and biosimilars alike. But they should be approached with caution and appropriate skepticism, especially when accompanied by claims of universality. And no company should let itself become so entrenched or dependent on a given approach that it cannot consider other promising ideas.
Connect and Learn in 2013
Explore these topics in more depth and network with others who are pushing the protein envelope next summer in San Francisco, CA, for IBC Life Sciences’ Next-Generation Protein Therapeutics Summit. Cross-fertilize ideas from different disciplines to help you turn promising new molecules into products. The 2012 program devoted three days (25–27 June) to these sessions:
Beyond Antibodies
Protein Engineering and Design
Intracellular Biologics and Alternative Delivery
Optimizing Properties of Next-Generation Biologics.
To be part of the June 2013 program — or simply learn more about it as you plan to attend — go to www.ibclifesciences.com/ProteinSummit
And in Europe, look for next year’s third annual Non-Antibody Protein Production conference from Informa Life Sciences. The most recent meeting was held in Prague (24–25 May 2012) and included discussion of purification platforms with case studies from UCB Celltech, Abbott, Ohio State University, Synthon, and Bayer Pharmaceuticals.
Find more information — and to download exclusive interviews with William Gillette (senior scientist at SAIC), Michel Eppink (head of downstream processing at Synthon), and David Bramhill (director of RCTech) — at www.informa-ls.com/event/nonantibody2012.
About the Author
Author Details
Cheryl Scott is senior technical editor of BioProcess International. Quotes not otherwise attributed are from personal correspondence.
REFERENCES
1.) Kelley, B. 2007. Very Large Scale Monoclonal Antibody Purification: The Case for Conventional Unit Operations. Biotechnol. Prog 23:995-1008.
2.) Ransohoff, T. 2005.. The Use of Disposable and Alternative Purification Technologies for Biopharmaceuticals.
3.) Gagnon, P 2012. Technology Trends in Antibody Purification. J. Chromatogr. A 1221:57-70.
4.) Gottschalk, U, K Brorson, and AA Shukla. 2012. The Need for Innovation in Biomanufacturing. Nat. Biotechnol 30:489-492.
5.) Williams, S. 2011.Affinity Platform Approaches for the Purification of Non-Antibody Bio-TherapeuticsFuture Technologies in Downstream Processing, London.
6.) Nelson, AL. 2010. Antibody Fragments. MAbs 2:77-83.
7.) Durocher, Y. 2012. Simple, Scalable and High Yield Method for High Level Production of Human Recombinant Interferon (L-11993), National Research Council Canada, Ottowa, ON.
8.) Roberts, SS.. Cytokine Research Proliferates Despite Growing Pains.
9.) Seely, JE, and RA Hart. 2012. Prior-Knowledge Assessments: Leveraging Platform Process Experience to Develop Targeted Process Characterization Strategies. BioProcess Int 10:12-19.
10.) Scott, C 2004. Classifying Vaccines: From Cowpox to the Cutting Edge. BioProcess Int 2:14-23.
11.) Ngai, J. 2011..
12.) Engeleka, M Accelerated Production of Subunit Vaccine Antigens Using SUMO Fusion Tags and Single-use Bioreactors, Life Sensors, Malvern.
13.) Pogorelc, D.. Cancer, 5 Ot
her Areas Focus of Vaccine Market.
14.).
15.) Summerford, C, and RJ. Samulski. 1999. Viral Receptors and Vector Purification: New Approaches for Generating Clinical-Grade Reagents. Nat. Med 5:587-588.
16.) Rosenblatt, B 2012. Meeting Regulatory Challenges for Cell-Based Therapies. BioProcess Int 10:S8-S11.
17.) 2008. Recovery and Purification: Q&A with Scientific Advisors. BioProcess Int 6:36-43.
18.) Scott, C 2012. A Decade of Chromatography. BioProcess Int 10:S32-S36.
19.) Low, D, R O’Leary, and N. Pujar. 2007. Future of Antibody Purification. J. Chromatogr. B 848:48-63.
20.) Barnard, GC. 2005. Integrated Recombinant Protein Expression and Purification Platform Based on Ralstonia eutropha. Appl. Environ. Microbiol 71:5735-5742.
21.) Detmers, F. 2010. Novel Affinity Ligands Provide for Highly Selective Primary Capture. BioProcess Int 8:50-54.
22.) Gagnon, P. 2008. The Emerging Generation of Chromatography Tools for Virus Purification. BioProcess Int 6:S24-S30.
23.) Gagnon, P. 2010. Monoliths Open the Door to Key Growth Sectors. BioProcess Int 8:20-23.
24.) Gagnon, P. 2010. Minibodies and Multimodal Chromatography Methods: A Convergence of Challenge and Opportunity. BioProcess Int 8:26-35.
25.) Gavin, D, and P Gagnon. 2006. Building Process Control into Chromatographic Purification of Viruses. BioProcess Int 4:22-30.
26.) Gronberg, A. 2007. A Strategy for Developing a Monoclonal Antibody Purification Platform. BioProcess Int 5:48-55.
27.) Hey, T. 2005. Artificial, Non-Antibody Binding Proteins for Pharmaceutical and Industrial Applications. Trends Biotechnol 23:514-522.
28.) Koide, S, A Koide, and D. Lipovsek. 2012. Chapter Six: Target-Binding Proteins Based on the 10th Human Fibronectin Type III Domain (10Fn3). Protein Engineering for Therapeutics, Part B. Meth. Enzymol 503:135-156.
29.) Malinowski, JJ. 1995. Production, Purification, and Crystallization of Human Interleukin-1P Converting Enzyme Derived from an Escherichia coli Expression System. Protein Sci 1:2149-2155.
30.) Proudfoot, AEI. 1990. Preparation and Characterization of Human Interleukin-5 Expressed in Recombinant Escherichia coli. Biochem. J 270:357-361.
31.) Ruigrok, VJB. 2011. Alternative Affinity Tools: More Attractive than Antibodies?. Biochem. J 436:1-13.
32.) Segura, MM. 2005. A Novel Purification Strategy for Retrovirus Gene Therapy Vectors Using Heparin Affinity Chromatography. Biotechnol. Bioeng 90:391-404.
33.) Sirko, A. 2011. Recombinant Cytokines from Plants. Int. J. Mol. Sci 12:3536-3552.
You May Also Like